by Mark Adamiak, Adamiak Consulting LLC, USA and Volker Leitloff, RTE, France
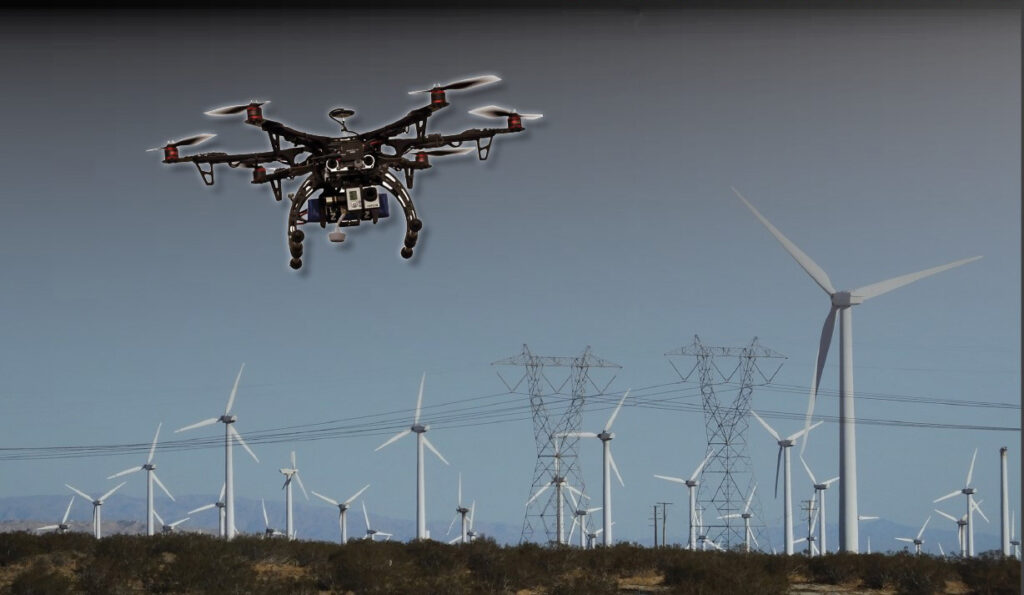
The structure of the electric power grid has started and will continue to change dramatically over the coming years with the implementation of distributed generation and new technologies to improve its efficiency and capacity. These poses many challenges, but also creates new opportunities. CIGRE WG B5.43 has elaborated these opportunities in the CIGRE Technical Brochure 629 “Coordination of Protection and Automation for Future Networks,” which was published in 2015. This document looks at a likely model of the future grid and its prospective components and identifies the challenges and requirements for protection and automation.
From these evolutions, potential solutions for protection and control, as well as follow-up investigations were proposed. Based on these points, requirements related to protection and automation of Future Networks were evaluated. This article describes the main findings of this work and, provides an update on some of the points after 6 years.
Power System: Everything Changes…
A first part of the Technical Brochure discusses the drivers for change of electrical networks. This concerns basic trends likely to have a significant impact on electrical networks, spanning from low voltage distribution to bulk transmission. These drivers include:
- The change of the generation pattern, in particular the increase of Distributed Energy Resources (DER) on the lower voltage levels and the increase of renewable energies, in particular wind farms and photovoltaic generation, which also can be connected to higher voltage levels
- Extended use of energy storage, adding new technologies like fly wheels and batteries, to the existing pump storage facilities. Generation trends change from predominantly large base load power plants connected at the transmission level to a mix of large dispatchable plants at transmission level along with a large amount of embedded generation. In this context, car charging and Vehicle to Grid approaches have to be added to the technological drivers identified in 2015
- Societal trends and governmental and international polices. Those will have a huge impact on the future grid and include line construction, disengagement from nuclear power or, on the contrary, use of new generation nuclear plants, regulations with multiple objectives, exchange clearing authority, energy exchange trade, Transactional Energy. and a growing market for system services
- Technological Drivers, including FACTS, SVC and HVDC devices providing solutions to some of the problems encountered in transmission networks such as mitigation of faults or enhanced transmission of power. Another important technological driver is communications enabling the gathering of information in order to make real time decisions based on an accurate knowledge of the state of the network. Many transmission substations already have access to adequate communication networks but many distribution substations and DER connected to LV or MV networks still have limited communication facilities
- The standard IEC 61850 for “Communication networks and systems for Power Utility Automation” will feature prominently in the Protection and Control solutions employed for the Networks of the Future – especially its ability to provide self-description and generic configuration. Originally developed for use inside substations, it has been extended to cover substation-to-substation and substation-to-EMS communication. It also addresses sampled values of current and voltage in the process bus. This facilitates the use of new technologies such as Low Power Instrument Transformers (LPIT)
- The use of station-wide and global time synchronization, the increased use of regional or zonal control systems, automation and protection schemes, and the constraints due to cyber security issues
- The emergence of 5G cellular communications which will make communication pervasive over the utility landscape
- Other drivers include energy storage development, zero energy homes, electrical vehicle evolution, home networks and other home automation developments, etc.
All of these drivers have a direct or indirect impact on virtually all protection and control functions. Some of them will require development of new or adaptation of existing PACS functions. In order to make a complete assessment of these impacts, it was necessary to develop a vision of the Future Grid, describing probable evolutions and characteristics of the Future Network. This includes the modification of the generation structure due to Distributed Energy Resources (DER) as mentioned above, the insertion of bulk transfer structures to accommodate massive integration of intermittent generators, significant evolutions of distribution grids due to DER and improved load management and other changes related to Smart Grid developments. The bulk transfer structures have been studied in the framework of the Global Energy Interconnection (GEI) studies and an evaluation of necessary standard developments to accompany these infrastructures is undertaken today. (Figure 1).
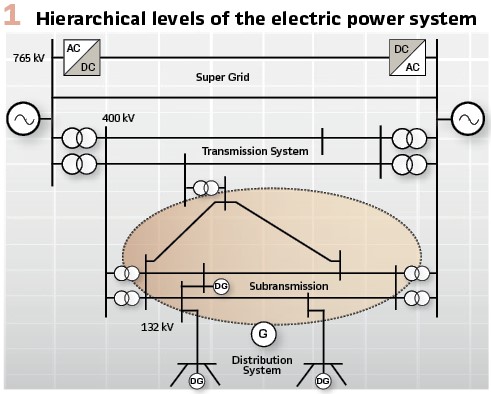
An example is the study for a European DC-Supergrid as it is contemplated amongst others by MEDGRID and the DESERTEC foundation. The DESERTEC-concept (Figure 2) was focused on the efficient development of solar resources in the Mediterranean area and the low-loss power transmission to the north of Europe by discrete HVDC-links. The creation of a DC-Supergrid composed of discrete (Multi-terminal) HVDC-links as the backbone of the 380 kV-three-phase system was envisaged. Since then, several studies have pushed further towards a real HVDC grid.
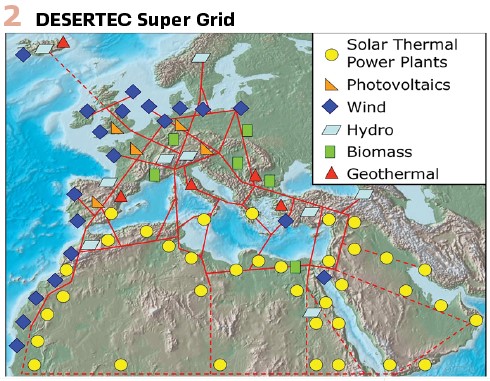
Increased use of Power Electronic Converters on all voltage levels is already under progress and other types of Energy Sources will appear in Future Networks such as Virtual Power Plants. Since they have an important impact on protection and automation, the main characteristics of the different types of energy sources coupled to the electrical network need to be considered. Other evolutions include increase of power flow on transmission lines and the use of innovative devices in the network such as Fault Current Limiters, Solid State Transformers, increases in underground networks, super conduction based equipment, Energy Storage Systems, and Low Power Instrument Transformers (LPIT). One of the main aspects on the future grid concerns the impact on lower/limited short-circuit current and transient behavior of inverter-based generation, with direct consequences for the sensitivity and selectivity of protection schemes.
… and the PACS must Adapt
An enhanced communication infrastructure will provide the path to the monitoring and automation of such equipment on the Future Grid. Specific challenges include fault protection in systems where the current is limited by the electronics or superconducting devices, micro-grid creation, management, and re-connection, voltage control/low-voltage ride-through of generation sources, multiple distributed energy resource synchronization/black start, higher variety of load currents and distributed automation. Expected technologies to aid in this evolution include migration to alternative voltage and current sensing technologies, wide-scale application of process bus technologies, general migration to IEC 61850 as the communication framework, significant expansion of wireless or cellular communication enabling all elements of the grid to become part of an Internet of Things (IoT).
On the Transmission side, the revolution of developing “situational awareness” through high-speed Synchrophasor measurements will continue to expand and will begin the clear migration to Dynamic Contingency Analysis, that is, a real time monitoring system that, upon detection of the first contingency on the grid, will run all possible N-1 additional contingencies and will identify the optimal control strategy to maintain system stability for all unstable contingencies identified.
Solutions impacting protection and control for augmenting the delivery of power will take many forms and may include:
- Line re-conductoring
- Dynamic Line Rating
- Video or sensor based thermal monitoring of power transformer and other equipment
- Migration to 6-phase on double circuit towers
- Use of Power Electronic based Transformers
- Superconductor based transmission lines and Fault Current Limiters
- Augmented voltage monitoring
- Significant expansion in the use of Static Var Compensation
- Thyristor Controlled Series Capacitors
There are also evolutions on the level of secondary equipment that will have a huge impact on protection and automation of the future networks. These evolutions include:
- Increase in processing and communication capabilities
- Higher functional integration
- Implementation/integration of standards such as IEC 61850 and CIM
- Performance increase in telecommunication technologies and evolution of the Internet of Things (IoT)
- Increased use of synchrophasors for grid monitoring and fault detection (High Impedance Faults, broken wire detection (already in demonstration)
It is obvious that automation of the operation of the grid will continue to grow in importance. What is to be noted is its growth as the “glue” that holds the Internet of Things together. As such, the standardization of automation tools, interfaces, and processes will be needed. There is just-completed report. in the IEC 61850 working group that defines standard interoperable logic for use in IEC 61850-based devices. It is expected to see mass deployment of standard logic for automation in the years to come.
Data Modelling of everything in the grid – using CIM and IEC 61850 – will grow very fast. There is already a convergence of CIM and IEC 61850 to facilitate such. The models will find use not only in protection and automation but also in system studies, asset management, and system operation (including dynamic control). Model enhancements will be required to include object dynamic performance information, for example, breaker operating times, fast valve performance, DER and inverter dynamics.
Impacts on protection and automation functions of several of the identified evolutions of the Future Grid are discussed in the Technical Brochure 626, including:
- Active Distribution networks
- HVDC links and networks
- Use of six phase networks
- FACTS
- Effect of Power Electronic Conversion
- Wind turbines
- Fault Current Limiter effect on Protection
- Superconducting lines.
Most of these aspects require however more detailed studies, and some of them have been covered by other CIGRE Technical Brochures. Some of these items are outlined below.
Protection of Active Distribution Networks
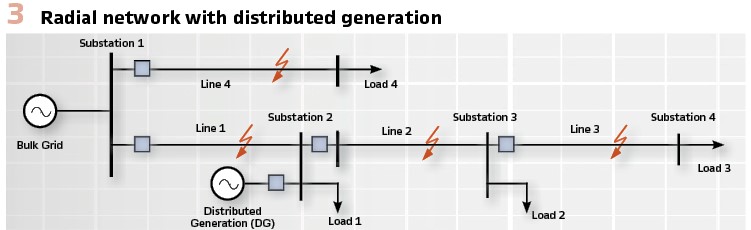
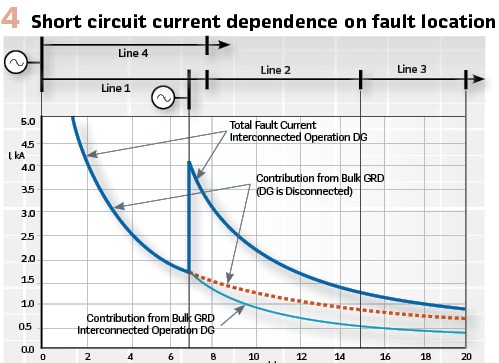
The use of Distributed Energy Resources has increased during last few decades and is expected to continue to do so. The connection of DER to the network changes power system conditions and can have a serious impact on protective relay operation. The IEEE 1547 standard will have to continue to evolve to address issues of protection and automation with increasing penetration of renewables. It is expected in future networks that all DER devices will be required to communicate – specifically, via IEC 61850.
Figure 3 shows a simple radial distribution network with DER connected at Substation 2. Dependence of short circuit current on fault location is presented in Figure 4. When the fault occurs at line 2 or line 3, total short circuit current is increased due to the connection of DER, however the contribution from bulk grid resources is reduced. When a fault occurs at the adjacent feeder (Line 4), reverse short circuit current is provided by DER.
Impact of DER can lead to:
- Loss of protection sensitivity
- Increase of fault clearing time (particularly due to cascading fault trips)
- Selectivity problems
For distribution networks, the use of closed loops instead of radial topology would provide an increase power quality/availability. This configuration does come with an impact on the protection scheme required for these networks. It is possible that the necessary upgrades of protection functions associated to the insertion of DER may address this possibility. (Figure 5).
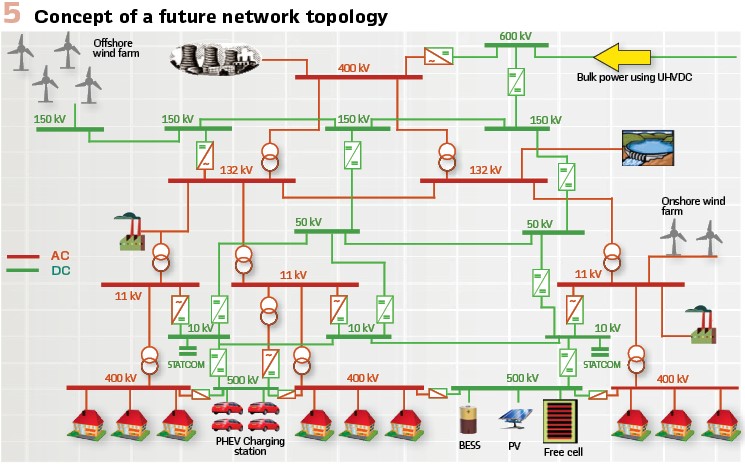
Protections Issues Related to HVDC Links
The Technical Brochure from CIGRE JWG B5/B4.25 (TB 484) addresses a list of phenomena related to LCCs (thyristor-based Line Commutated Converters) DC links which interact with the protections of the AC network. The identified issues are:
- Impact of harmonics created by converter poles online and transformer protections
- DC ground loop currents may cause saturation in power transformers or current transformers with adverse effects on AC protection
- Commutation failures of the converter poles may cause DC currents on the AC side. This may lead to CT / VT saturation and / or to protection system malfunctions
- Discharge current of DC cables after interruption of DC operation may cause saturation in power transformers or current transformers with adverse effects on AC protection
- Limitation of fault current infeed by converter poles. This may lead to situations where AC protection does not pick up or does not trip due to low fault current
- AC voltage distortion as a consequence of non-symmetric DC operation of the link may have adverse effects on AC protection
- Fast adjustment of converter parameters in case of AC or DC fault. This may cause transients leading to untimely trips or erroneous fault direction indication by AC protections
- DC pole commutation failures may cause transients leading to untimely trips or erroneous fault direction indication by AC protections
Depending on the constraints of available transmission corridors and existing lines, utilities may decide to build HVDC lines in the same corridor or even on the same support used by existing AC lines. Some approaches allowing use of an existing line either as AC or as DC line in case of converter pole unavailability are also possible. In this case, faults between HVDC and AC circuits can occur, and this eventuality has to be taken into account in the HV design and in the protection system of both systems. Depending on the grounding of the two networks, the resulting fault current may vary over a large range. It may be small in case of ungrounded or compensated AC systems or it may be greater than the phase-to-phase short-circuit current of one of the systems. This has implications both for the AC and for the DC protections.
In addition to bulk HVDC transmission networks, the appearance of local LV DC networks (e.g. limited to an area or even a building) is possible.
Power Electronic Converters
Many DER devices are interfaced to the distribution network by converters and/or devices based on Power Electronics. On MV and LV levels, they are normally equipped with loss of mains protection, but high DER penetration raises particular problems in distribution networks. In this context, improvement of islanding detection for networks with a high penetration of converter based DER is required.
Renewable Energy Sources (RES) with higher power can be connected to the transmission systems using Power Electronic Conversion. In this case, the connection point and the connection conditions are easier to supervise because there is a dedicated feeder.
Many of the power converters use frequency tracking to adapt to the frequency of the interconnection. This is problematic when trying to create an island from a deadline. Algorithms and systems will have to be developed to perform this function. Additionally, all of these devices are current limited from a short-circuit current perspective and may have an option of only contributing positive sequence current. Both features have impacts on the protection, automation and service restoration. An example of the connection using Power Electronics is the connection of Wind Turbine Generators (WTG). Figure 7 shows as example WTG type IV.
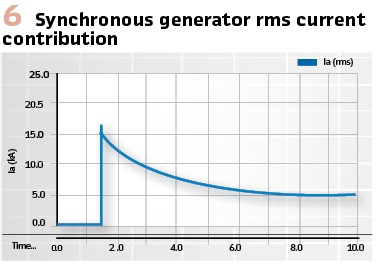
The connection of DER brings a great change to the configuration of the utility distribution network. It is expected that most DER will be installed on rural networks; however, future Smart Buildings may also be able to inject power into the network.
Comparing WTG types, Figure 6 shows a synchronous generator short circuit current contribution. There are major differences in the behavior of the different types of wind turbines (eg. synchronous versus doubly fed generator), with consequences for the associated protection and control functions.
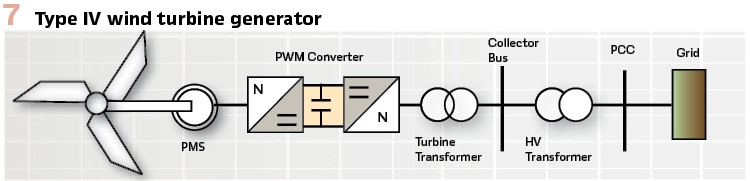
Full converter contribution depends on the converter behavior before short circuit. Most converters cannot supply important currents under external fault conditions; usually no more than 1.2 to 1.5 times their rated current of the converter. For that reason, it can be modelled as a synchronous generator with the option “Limit Maximum Phase Current” activated. In this case, fault detection schemes using overcurrent principles that are universally applied, are not usually effective. Wind turbines units that use this technology must rely on other methods such as abnormal voltage or frequency sensing to detect faults on the area power system.
Developments of PACS
In addition to the adaptation of the PACS to changing grid operation, condition and equipment as outlined above, the PACS itself is undergoing profound changes. This includes:
- Coordination of Protection and Automation for Future Networks and Evolutions of protection functions for different voltage levels and applications including extended use of Adaptive Protection and Control and Protection in networks operating under islanded conditions
- Evolution of Substation Architectures, including deployment of process bus and station bus, functional integration and decentralized Substation Automation and Control Systems
- Evolution of Communication Technology, including Wide Area Communications, Communication interface with customers
- Cloud Computing
- Internet of Things (IoT)
- Cyber Security
- Application of Synchrophasors
- Use of IEC 61850 and its extensions
- Fault Location
- Application of System Integrity Protection Schemes (SIPS)
- Use of Metering – especially at the DER level
The expected evolutions of these functions, partially enabled by the progress of communication and computing technologies, is discussed in TB 629.
Conclusions
Electricity has become one of the most essential commodities in the operation and maintenance of society and it is clear that it will only become more important in future networks. As an essential commodity, the need for abundant, reliable, and clean energy is going to drive the discussion for the next evolution of the electric power grid.
The paths to the power grid of the future are coming into focus and offer many advantages in the design, build, and operation of future networks. It will be up to the next generation of utility managers to decide which paths to follow and when. Explorers will be needed to lead the way. As they did in the past, protection and automation systems will support network evolutions. Due to the progress, which is being made in the secondary technology, they will also be able to provide valuable and substantial contributions to the design and operation of future networks.
Changes in the power grid will be driven by technological innovation, societal trends (e.g. Green energy, etc.), and governmental policies, as discussed above. The obvious result of these drivers is going to be the exponential expansion in renewable energy generation (mostly inverter-based), the emergence of energy storage, and the inter-networking of all elements in the grid. It is expected that the addition of these elements will be linked through DC networks. The vision is that DC will run in parallel with AC links and that AC or DC “supergrids” will be implemented over various wide geographic areas.
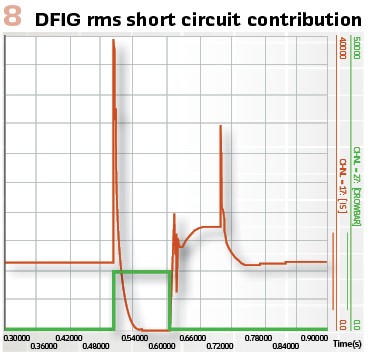
On the other end of the network, the consumer will also be playing a different role since all aspects of energy usage in the home will be monitored and controllable – Load as a Resource will grow in importance. In parallel, the electric car will become an important element in the load and generation balance on the grid.
These evolutions will bring challenges and require adaptation and new concepts in the protection and automation on all network levels, some of which have been outlined in this article. the probable evolution of electrical networks in the next 2-3 decades, until 2030-2040.
Biographies:

Mark Adamiak is an independent consultant for the electric power industry. Mark started his career in the utility business with American Electric Power (AEP) and in mid-career, joined General Electric where his activities have ranged from advanced development, product planning, application engineering, and system integration in the Protection and Control industry. Mr. Adamiak is an original member of the IEC61850 WG, a Life Fellow of the IEEE, a registered Professional Engineer in the State of Ohio and a GE Edison award winner. Mark was the Principal Investigator for the EPRI IntelliGrid project to develop a reference architecture for the Smart Grid. In 2012, Mr. Adamiak was elected to the US National Academy of Engineering.

Volker Leitloff has a Dipl-Ing. degree from the university of Stuttgart, Germany and a Dr. INPG degree from the Institute National Polytechnique de Grenoble, France. From 1994 to 2002, he was with the R&D Division of Electricité de France. Since 2003, he has been with the French Transmission Network Operator RTE where he works on protection of transmission networks and substation control. He is chair of IEC TC38 and CENELEC TC38 (Instrument Transformers), convenor of IEC TC95 WG2 Digital I/O of protection IED, past convenor of CIGRE WG B5.06 on DSAS Maintenance and actual or past member of IEC TC38WG37, TC38WG47, TC95MT4 and CIGRE WG 34.10, B5.10, B5.13, B5.43, B5.53, B5.64, B5.69 and the TAG and SAG of CIGRE Study Committee B5.