Building microgrids for enhancing energy resiliency, affordability, and sustainability
by Mital Kanabar, GE, Canada, Nathan Dunn, GE, USA, and Steve Halford, GE, USA
The world is in the midst of change. More than ever before, there is growing concern over the resiliency and reliability of power. Increasing extreme weather events, renewable energy integration, and frequent, unpredictable costs are just some of the challenges that modern power grids are facing. Energy demand is transforming. Energy goals are shifting. Decarbonization strategies are escalating. Digitization is revolutionizing the game. And when the power goes out, what do we do?
The current energy landscape – combined with consumer demand for solutions that provide increasing reliability, resiliency, sustainability, energy independence, and cost reduction – have led to a growing demand for microgrids.
What Is a Microgrid? A microgrid is a local, self-sufficient energy system that typically serves its own specific network, such as a utility, correctional facility, military base, hospital complex, or academic campus. The U.S. Department of Energy Microgrid Exchange Group defines it as “a group of interconnected loads and distributed energy resources (DERs) with clearly defined electrical boundaries that acts as a single, controllable entity with respect to the grid. A microgrid can connect and disconnect from the grid to enable its operation in both grid-connected or islanded mode.” In other words, a self-sufficient island within the larger grid ocean.
While microgrids generally operate while connected to the grid, they can operate on their own in the event of a crisis. Further, depending on how a microgrid is managed, it can essentially run indefinitely. Ultimately, a microgrid has the capability of keeping electricity going when the power otherwise goes out.
Microgrids are not a new thing. Hospitals and military bases, for instance, often install microgrids to mitigate their risk of power outages; as essential services, they cannot tolerate interruptions and continuous power is critical. While the concept and implementation aren’t new, the opportunity now is enabling the energy transition with innovative solutions utilizing renewable energy.
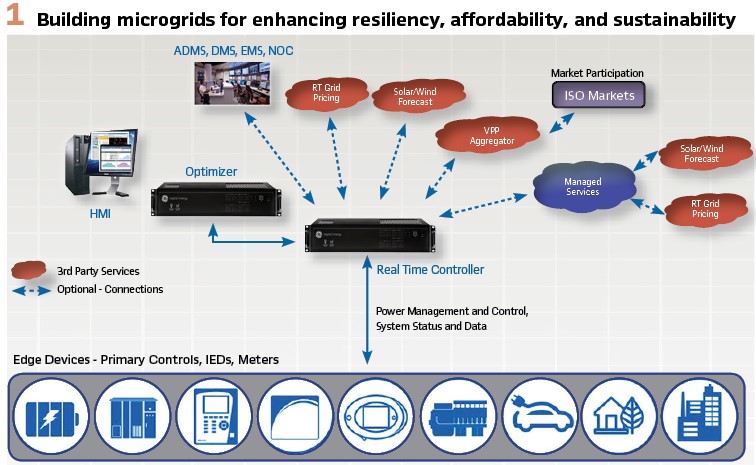
Why Microgrids?
To meet carbon neutrality goals, corporations have started to implement sustainability programs at their facilities. When implemented effectively, these programs involve the integration of onsite renewable energy. Onsite renewable integration varies across a broad spectrum but, when leveraged as part of a microgrid, can provide additional benefits beyond sustainability including energy savings, reliability, resiliency, and revenue generation. These benefits are obtained through the integration of advanced control and optimization platforms that are tailored and configured to meet the facility’s objectives, including:
- Increased reliability enhancements through real-time detection of unstable system behavior and isolation
- Improved resiliency by providing a system that can self-isolate from the affected grid and continue to support its loads independently for desired periods of time
- Energy cost reduction through a solution that can efficiently manage and optimize your energy resources based on real-time energy market prices, operational costs, and energy resource mix
- Increased revenue by enabling your system to provide ancillary services to the grid such as frequency control, reserve capacity, and demand response
- Energy security by effectively controlling, monitoring, and optimizing your local energy assets, reducing your need to rely on a centralized supply
- Increased percentage of renewables integration through an energy management solution that supports the transition to new renewable energy targets and policies
- Reduced emissions from on-site generation sources through the optimal dispatch and management of your energy resource mix
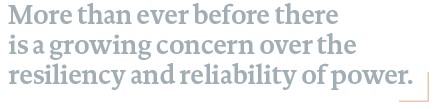
An industrial facility’s primary goals are centered around production. A key factor that can affect production output is down time caused by an interruption or instability in the supply of energy. Historically, the electric grid is a very secure source of power, enabling most companies to reduce their risk with the deployment of standby gas generation. However, standby generation may no longer be adequate as the percentage of intermittent renewables on the grid continues to increase, threatening the stability and reliability of the electrical network. This is happening while industrial costs of down time continue to rise. In addition, as many corporations are moving toward carbon neutrality and a net-zero manufacturing footprint, these initiatives could require the deployment and integration of onsite renewable energies to offset some of the emissions of electrical consumption from the electric grid. The intermittent nature of onsite renewable sources presents a challenge for a facility’s network stability, particularly when disconnected from the electric utility grid. Microgrid with renewable energy sources can be configured to optimize, control, and balance a facility’s power generation and loads based on the customer defined goal.
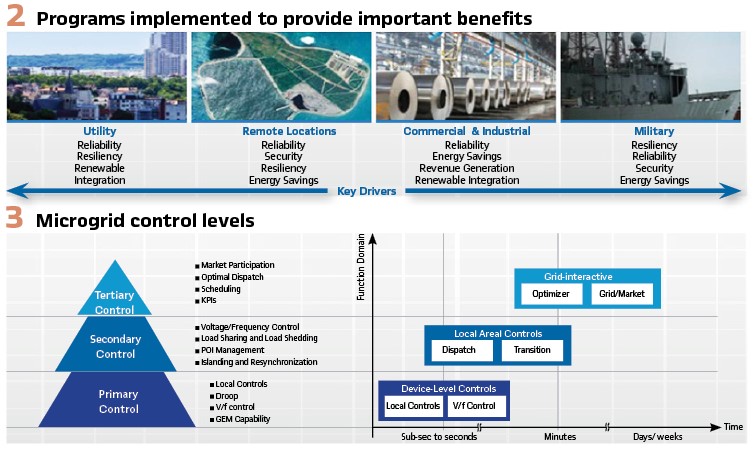
Microgrid Control Levels

A microgrid control solution is comprised of two parts: the real-time secondary controller and the tertiary level optimizer. The real-time secondary controller is responsible for the reliability of the system through high-speed communication, control, and automation. The tertiary level optimizer is responsible for the customer-desired outcome by producing a day-ahead operating or optimal dispatch plan. This plan is tailored based on the facility’s desired outcome or mode of operation such as economics, reducing emissions, maximizing time of life, maximizing renewables, or peak shaving. These two systems work together to provide the facility an optimal solution to manage the system in grid-connected and islanded mode, maintaining reliability, resiliency, and customer outcome. (See Figure 3).
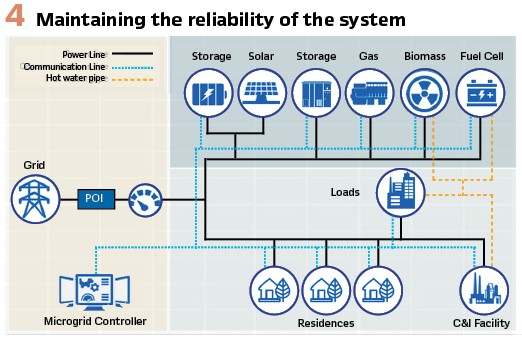
The microgrid real-time controller is responsible for maintaining the reliability of the system, while following the optimal dispatch plan generated by the optimizer. This is achieved through communication with various intelligent electrical devices (IEDs) and the onsite distributed energy resources (DERs). (See Figure 4).
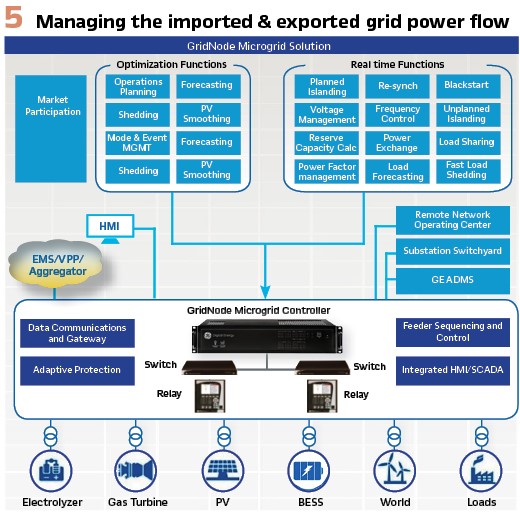
DERs are any type of onsite energy delivery asset, such as gas generation, a battery energy storage system (BESS), or renewables like solar or photovoltaics (PV) and wind. The microgrid controller dispatches setpoints and command structures to the DER assets and loads to manage the amount of grid power flow imported or exported based on the optimized dispatch schedule (Figure 5).
The optimal dispatch schedule should incorporate grid electricity prices, renewable generation forecasts, facility load forecasts, individual asset information and limits, as well as the chosen method of optimization. In addition, when called upon, the microgrid controller can be configured and integrated into energy markets to provide grid-related services in the form of demand response, voltage support, and frequency regulation, driving additional revenue for the facility. (See Figure 6).
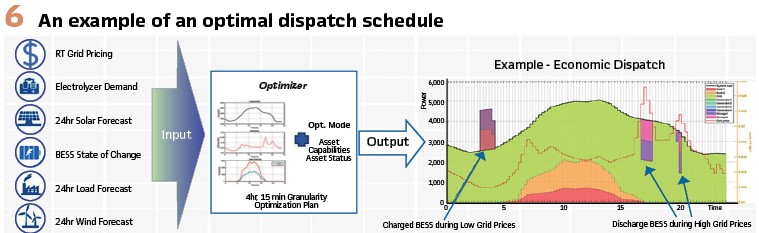
Day-ahead generation and load forecasting help determine the most effective time of day to utilize each generation asset. This plan can also incorporate dynamic load management to reduce or shed certain loads based on energy costs or to maintain the reliability of the system operations. However, when there are short-term events like wind gust, cloud cover, or large load fluctuations that affect the reliability of the system, these events are managed by the real-time controller. The real-time controller is in continuous communication with the optimizer, enabling the next dispatch schedule to make any needed adjustments.
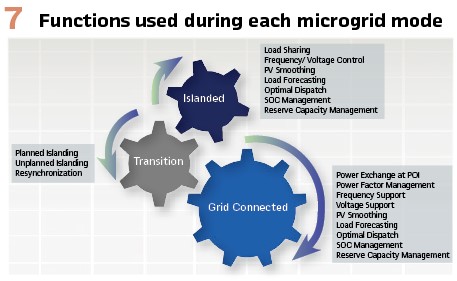
A microgrid is required to operate in grid-connected and island mode as well as manage the transition between the two operating modes. There are certain functions that are utilized during each microgrid mode of operation to maximize the reliability and resiliency of the system. (See Figure 7).
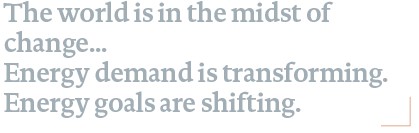
When a reliability issue is present on the grid, the microgrid is expected to transition to islanded mode of operation, while maintaining the reliability and stability of the microgrid network. To maintain this stability, proper consideration must be given to control functionality and design to account for functions such as fast load shedding, unplanned islanding, voltage and frequency control, load sharing, and reserve capacity management. In addition, the microgrid controller is responsible for assigning the grid-forming DER for maintaining the voltage and frequency of the islanded system. Other DERs operate in grid-following mode, contributing to the voltage and frequency control through secondary control loops to maintain the voltage and frequency at nominal values, after any deviation.
Microgrid Development Stages
During the design phase of the microgrid project, proper consideration should be given to the type of DERs being utilized, DER sizing, production operations and type, environmental regulations, and energy incentives. These considerations should be coupled with the facility’s objectives such as reliability, resiliency, energy savings, and/or sustainability as well as the facility’s current load profile and energy rates. Together, these inputs help provide a design that not only meets the facility’s goals, but also provides a solution that is economical and avoids oversizing.
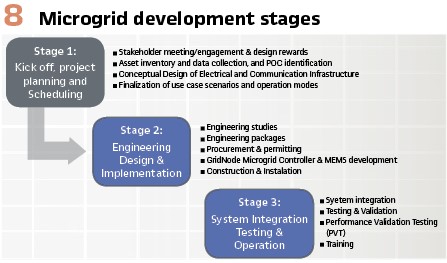
Once the determination has been made that a microgrid aligns with needs of the facility, the secondary part of the design process consists of designing the most effective communications and controls architecture for the solution. This architecture must be designed to enable the solution to provide the functionality and benefits required by the customer. Key considerations in this design include redundancy, speed, utilization of industry standard protocols, and cybersecurity. Frequently, the architecture will consist of a microgrid real-time controller and optimization server, which communicates to the various DER controllers, protective relays, meters, building management system (BMS), and plant SCADA. In addition, the controller may be connected to external sources for weather forecasts, real-time energy pricing, or energy market interfaces for demand response or frequency services participation.
Microgrid Scenario Simulation and Results
Let’s consider an electric grid-connected industrial-based microgrid that consists of the following assets:
- Two BESS rated at 1MW/2MWhr each
- Two PV plants rated at 1.5MW and 0.75MW
- Four gas generators rated at 2MWs each
- Three non-critical loads that can be shed
In this study the external inputs, facility load profile, time of day grid price, and solar forecasts were not changed for each of the three test scenarios to highlight how a customer’s desired optimization methodology and mode affect the 24-hour dispatch plan as well as highlight the optimizer’s ability to dispatch the system for various outcomes and use cases.
Scenario 1: Economic Dispatch
- Economic dispatch is an optimal dispatch mode with the goal of minimizing the cost of electricity for the customer. The optimizer must, at a minimum, consider the following information for this scenario:
- Grid prices based on a time-of-day schedule
- Gas generator operating costs including fuel costs, maintenance costs, efficiency curves, emissions costs, as well as loading and ramping times
- BESS – SOC bands, efficiency, charge and discharge rates
- PV – effective generation costs
- Loads – reduction costs
Cost is based on:
- Grid pricing – time of day pricing schedule
- Generator – fuel cost, maintenance cost, efficiency curves, emissions costs, loading and ramping times
- BESS – SOC band, efficiency (charge/discharge), max charge and discharge capability
- Renewable DER – effective generation cost
- Loads – priority, reduction cost, if they can be shed
- Real-time DER asset status
- Reserve margin requirements
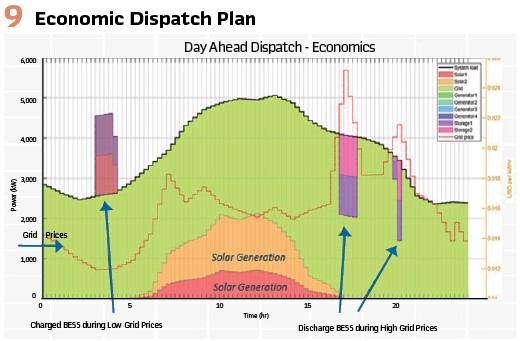
The 24-hour economic dispatch plan generated shows how the optimizer plans to leverage the BESS to charge during low grid price times and discharge during high grid price times. In addition, all four generators remain off due to the cost of generation. (see FIgure9).
Scenario 2: Peak Reduction
In a peak reduction scenario, the optimizer will look to dispatch the units in the same economic dispatch mode while limiting the grid import based on the user-configured data. The optimizer utilizes all the same inputs as in Scenario 1, with the addition of the following:
- Grid limit equals 1MW from 2:45 pm – 10:30 pm
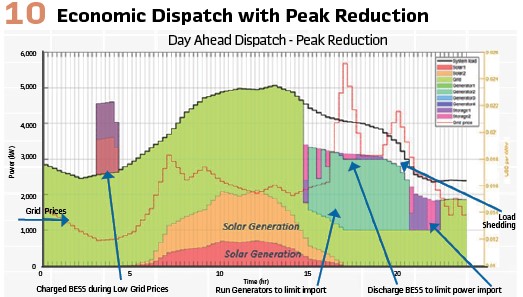
The 24-hour economic dispatch plan generated shows how the optimizer plans to leverage the BESS to charge during low grid price times and discharge during the timeframe where the grid limit is applied. In addition, the optimizer sheds non-critical loads as well as starts a gas generator to support the facility’s critical load demand that is greater than the 1MW grid import, BESS discharge, or renewable generation available. (See Figure 10).
Scenario 3: Planned Islanding
In a planned islanding scenario, the optimizer will look to dispatch the units in the same economic dispatch mode while removing the grid import based on the user-configured data. The optimizer utilizes all the same inputs as in Scenario 1, with the addition of the following:
- Planned island between 2:45 pm – 10:30 pm
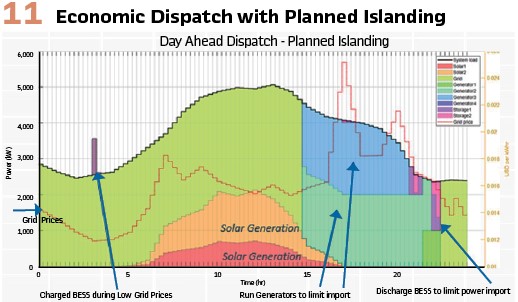
The 24-hour economic dispatch plan generated shows how the optimizer schedules the islanding of the system and disconnection from the grid power in the dispatch plan. The physical islanding of this system is the responsibility of the real-time control functions as part of the systems operations. Furthermore, this scenario required the start-up and operation of multiple generators to maintain the facility during the islanded period. see Figure 11).
The optimizer plan is based on the objective plus:
- Grid pricing – time of day pricing schedule
- Generator – fuel cost, maintenance cost, efficiency curves, emissions costs, and loading and ramping times
- BESS – SOC band, efficiency (charge/discharge), max charge and discharge capability
- Renewable DER – effective generation cost
- Loads – priority, reduction cost, if they can be shed
- Real-time DER asset status
- Reserve margin requirements
Summary: Grid reliability and resilience are important from a regulation’s perspective, but also support one of the key aspects of the World Energy Trilemma – Energy security, affordability, and sustainability. This article highlights how a properly designed microgrid with optimization capabilities enables a facility to meet its sustainability initiatives and integrate renewable assets onsite while providing the added benefits of reliability, resiliency, and energy savings.
The optimizer and controller can be tuned to enable multiple outcomes for the facility and provide the flexibility to support future changes the facility may encounter. With three different scenarios, it is important to note that the optimizer generates key performance indicators as well as provides the outcome of the optimization cases to be previewed and compared prior to implementing/deploying.
This capability provides proper visibility to the operations and enabling a case or implementing an optimization change.
Biographies:
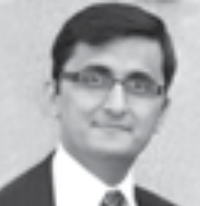
Mital Kanabar is Chief Applications Architect & Office of Innovations Leader at Grid Automation, Grid IQ Center, in Markham, ON, Canada. Mital has 15+years of industrial R&D experience and received his Ph.D. from the University of Western Ontario, Canada. Mital holds 14+ international patent applications, contributed to 50+ journal/conference papers, and five industrial magazine articles. He is also serving as a Chair of IEEE PSRC WG H47 (Digital substation); Vice-Chair of WG C40 (PDC standard); and Secretary of WG H45 (Centralized P&C); and participates in various technical working groups at IEC/CIGRE/NERC, Linux Foundation Energy (LFE), and other industrial technical community groups.
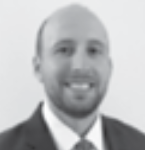
Nathan Dunn is the Global Grid Modernization Services Product Manager for GE’s Grid Automation business. He is responsible for leading the product line for Distribution and Microgrid Solutions as well as leading the Grid Modernization Center of Excellence team. This involves working across GE teams to develop, execute, and lead Grid Automation’s strategy for products, solutions, and services. Nathan started his career at GE as a Field Engineer and held positions inside Operations and Services Management prior to joining SEL as a Key Account Manager and Regional Sales Manager. Nathan joined GE in 2019 as the Global Business Development Services Leader for Grid Automation. Nathan earned a B.S. in Electrical Engineering Technology from the University of Pittsburgh and an MBA from the University of Maryland.
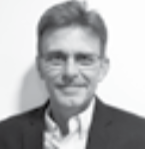
Steve Halford is the Microgrid Leader for GE Grid Solutions, bringing together multiple GE businesses to deliver reliable, efficient, and sustainable Microgrid systems to customers within North America. Steve has over 25 years of electrical power experience focusing on power quality, energy efficiency, power system design, and microgrid planning, design and development. Steve was the previously the Director of North America for Dranetz Technologies, and Traction Power, Mission Critical, and OEM Business Development Manager for SEL prior to joining GE in 2018. Steve serves as a co-chair to the IEEE Traction Power Committee for substation protection and control and participates in an advisory role on different NFPA standards groups and is also a volunteer firefighter and serves as his county lead safety trainer on energy storage systems for first responders.