by Andre Smit, Quanta Technology, USA
As distribution feeder automation (DFA) systems evolved, communication system advances significantly improved the speed at which power restoration actions can be performed. This improvement in restoration times has a positive impact on reliability KPIs.
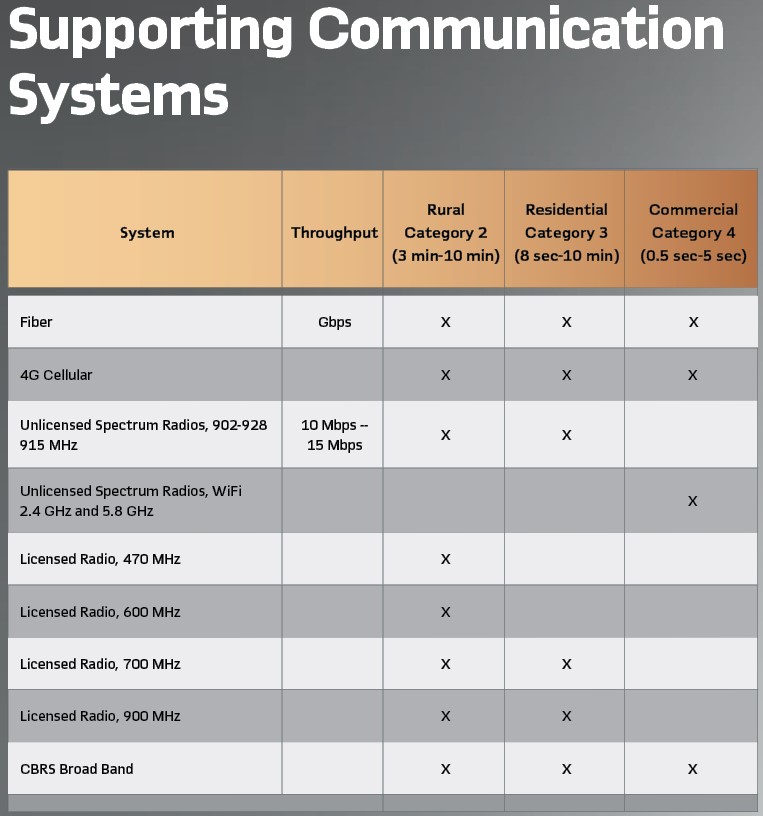
DFA Categories based on Performance
The first category of feeder automation is depicted in Figure 1. One is where the operator decides how to isolate and restore power based on loading and fault information received from the field.
Two centralized applications determine and recommend switching actions to the control center operator.
Three is automated switching executed by smart applications.
The fourth category includes protection and DFA. These systems will use protection functions to isolate the fault from the distribution network, identify the location of the fault and perform isolation, restoration, and test actions. These systems are decentralized. Protection devices that control the switchgear are used for DFA. The devices will automatically open or close the switchgear.
The DFA categories have different requirements for communication systems that will support the applications. DFA systems require two-way communication.
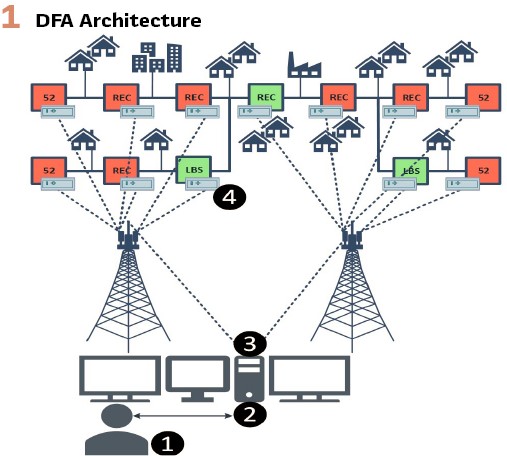
Distribution Feeder Automation Systems Performance
Distribution feeder automation categories:
1. Manual control room switching
2. Application recommended manual control room switching
3. Application automated control room switching
4. Application automated field device switching and protection
The operating characteristics of the automation systems provide a basis for selecting a communication system.
The switching actions of a DFA to perform the fault isolation and power restoration are referred to as Fault Locate Isolate and Service Restoration (FLISR). The performance of the different system’s FLISR switching actions is ranked in Table 1. Communication systems must support the DFA system’s performance. Processing information by applications takes a fraction of the time. Time is primarily lost in data exchanges. DFA systems need status, fault, and loading information for processing.
DFA Communication Protocols
DNP 3 is a master-slave protocol suited for centralized solutions.
Proprietary protocols are used to achieve better latencies.
IEC61850 provides Generic Object-Oriented Substation Event (GOOSE) messages that are small, broadcasted data packages that can contain status, fault, and loading information. GOOSE can perform high-speed data transfer between devices. The GOOSE messages must be used on IP-based communication systems.
Communication Systems Supporting DFA
Traditionally utilities used wireless communication to the large numbers of field switchgear in a DFA system.
Wireless Radio
Radio Fundamentals: As depicted in Figure2, the radio receives digital bits of information. The baseband transmitter, through modulation, converts the data into a digital signal within the channel frequencies. The digital signal is then passed to a digital-to-analog converter. The mixer converts the analog baseband frequencies to the carrier frequency, making it suitable for transmission. The analog filter avoids interference from devices operating over adjacent channels.
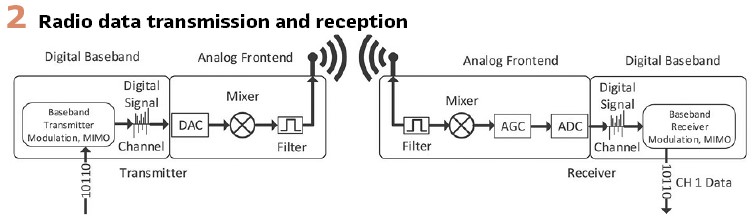
The receiver in Figure 2 receives the carrier frequency, filters the signal, then converts it back through the mixer to the bandwidth frequencies. Automatic gain control scales the analog signal from the antenna to ensure that the signal from the antenna is within the desired voltage range. The signal is then converted to a digital signal in the analog-to-digital converter. The signal is then demodulated in the baseband receiver back to data bits.
Radio Spectrum: The radio spectrum is the part of the electromagnetic spectrum with frequencies from 0 Hz–3,000 GHz (3 THz). The generation and transmission of radio waves are strictly regulated. The Federal Communications Commission (FCC) allocates different radio spectrum parts for transmission technologies, applications, and services.
Signal Strength Received Signal Strength Indicator: The Received Signal Strength Indicator (RSSI) measures how well a receiving radio can hear a signal from a transmitting radio. It helps determine if the radio has enough signal for a good wireless connection for data transfer.
Carrier Frequency: Carrier frequency is a frequency that is modulated within a radio to transmit data. More data can be transported at higher frequencies and amplitudes.
Channel: The channel is the frequency band that radio can use in modulation to form a signal for transmission.
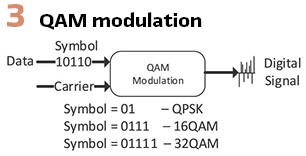
Modulation: Modulation level provides the best indication of a radio data link health. Modulation is used by radio to convert a radio wave into a signal representing data it transmits to a receiving radio. The receiving radio demodulates the radio waves back into data, as shown in Figure 6. Digital information is easy to modulate as it is represented by a 1 or 0 and is easy to encode. Quadrature amplitude modulation (QAM) is commonly used in modern radios.
QAM is a signal in which carriers are modulated and combined, increasing the data that can be transmitted and received. At the input of the modulator is digital data processed by groups of bits or symbols, as depicted in Figure 3. The other input receives the RF carrier signal.
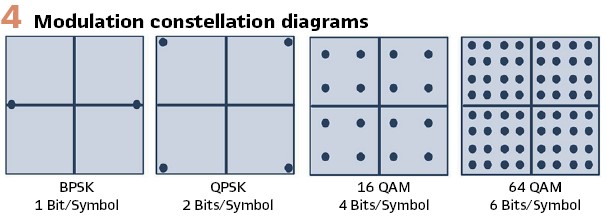
These input signals are combined in the modulator that combines the signals that control the amplitude and phase of the resulting modulated output signal. The length of the symbol determines the QAM modulation depth and the maximum number of symbols. The symbols can be mapped into constellation diagrams, as seen in Figure 4.
The radio will select the modulation BSPK, QPSK, 16 QAM, 32 QAM, 64 QAM, 128 QAM, or 256 QAM based on the interference it measures. More data can be transported at the higher modulation levels.
Interference: Interference is measured as the signal-to-noise ratio (SNR). The radio measures the signal it receives and the noise it receives. It then determines the modulation level. The radio must measure a high signal-to-noise ratio for a higher QAM modulation.
Throughput: Throughput is the number of bits per second at which a wireless or wired link can transfer data. (e.g. 100 Mbps). Radio manufacturers specify the physical rate, as shown in Figure 5, but the physical rate consists of user data and protocol overhead. The radios use the protocol overhead data to exchange information that facilitates successful communication. The user throughput is a fraction of the physical rate. Available Modulation and Coding Scheme (MCS) Index Charts provide what throughput can be expected based on the radio settings.
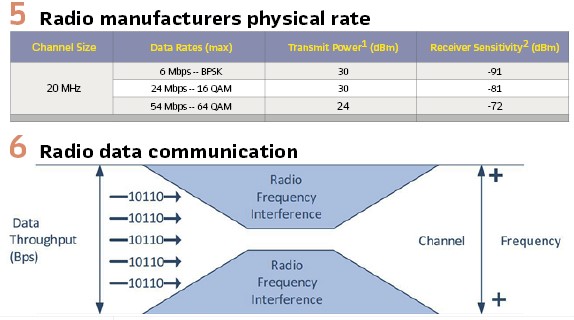
The basic concept of understanding radio data communication is that the data throughput is highly dependent on a radio link and interference, as depicted in Figure 6.
Data Packet Size: Smaller data packets are processed more efficiently and, thus, more reliable. Fragmentation is used for large data packets through a process that breaks data packets into smaller fragments so that the resulting pieces can pass through a link with a smaller maximum transmission unit (MTU) than the original packet size. The receiving host reassembles the fragments. Fragmentation can lead to packet loss and excessive retransmissions.
FDA Radio Systems: A select few radio systems are used in a DFA. Lower frequencies can be transmitted over longer distances, as illustrated in Figure 7. The lower frequencies can deliver less data than higher-frequency radios. Low frequencies require less tower infrastructure than high-frequency radio systems and distances and are less affected by physical obstructions between radios.
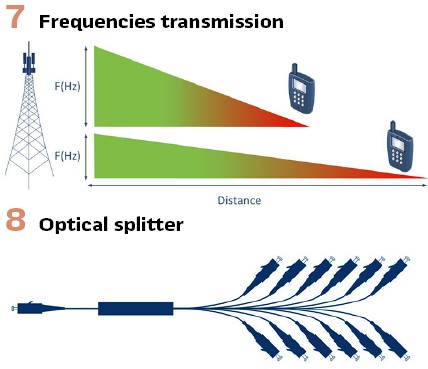
Unlicensed Spectrum Radio: In the United States, we have 902 MHz–928 MHz low frequency, 2.4 GHz, and 5.8 GHz high frequency (commonly referred to as WiFi). The industrial, scientific, and medical band (ISM) is at 915 MHz.The unlicensed radio spectrum is busy, and interference could impact throughput.
Spread-Spectrum Radio: Spread-spectrum radios are popular for creating wireless communication links. These low-cost devices provide strong links ranging in throughput from 10 kbps–200 kbps and distances of 3 mi–50 mi, depending on the radio and operating conditions. Spread-spectrum radios spread the normally narrow-band information signal over a relatively wide band of frequencies, making communication highly immune to noise and interference sources.
Meshed Radio: Meshed radios are connected in a mesh topology. The networked mesh radios are reliable and offer redundancy. When one node can no longer operate, the rest can still communicate directly or through one or more intermediate nodes. Throughput is reduced, and latency is increased through each radio node that the data packet must travel.
Licensed Radio: Licensed radio frequencies are available from advanced metering infrastructure (AMI) and Distribution Automation system providers. Radio system providers acquire frequencies from the Federal Communications Commission (FCC) for commercial and industrial use. The available spectrums are:
- 470 MHz, a spectrum owned by Aclara for AMI use
- 600 MHz, a spectrum owned by T-Mobile and spectrum speculators used for private long-term evolution (LTE) or 5G
- 700 MHz, a band owned by Access Spectrum or Select Spectrum
- 900 MHz, a spectrum owned by Anterix, will be used for private LTE networks
- There is a spectrum in 900 MHz used by Sensus for DA and AMI
These communication systems have excellent latencies and can support all categories of distribution feeder automation. Providers manage the congestion of these private networks to ensure clean and reliable communication.
Lightly Licensed or Managed Spectrum: This is the Citizens Broadband Radio Service (CBRS) 3550 – 3700 MHz band. The FCC established CBRS for public and commercial use. The FCC certified Google, CommScope, Federated Wireless, and Sony to operate as Spectrum Access System (SAS) administrators in the 3.5 GHz (WiMAX) band.
General Authorized Access (GAA) licenses are on a shared basis, available using a SAS database. The SAS is a cloud-based service that manages the wireless communications of devices transmitting in the CBRS band to prevent harmful interference to higher-priority users. A CBRS device (CBSD) needs authorization from the SAS provider before it starts to transmit in the CBRS band.
CBRS digital IP-based radio routers provide reliable data connectivity for critical applications that require stability, availability, and security.
The point-to-multipoint (P2MP) architecture requires base stations on towers at a minimum of 200 ft high. The radios are near the line of sight and can reach distances of 10 miles on average.
Cellular
Private cellular networks are available from several providers and are widely used by utilities for various services and applications. The cellular communications systems have excellent coverage and penetration, except in rural areas. The cellular providers can provide private networks for utilities that are not connected to the public internet network. The providers also offer priority services to utilities.
The cellular options are subject to constant change as these networks are extensive and have many devices, servers, applications, and routers that could affect services daily and must therefore be constantly monitored for availability and performance. Automation systems must be designed to overcome short communication interruptions.
Deployment is simple, requiring a machine-to-machine (M2M) data plan and a cellular modem with an antenna communicating with the field control device. Cellular modem configurations can be standardized. The provider maintains the system except for the cellular modem. There are data charges per modem per month.
Fiber Communication
Fiber communication systems are the most desirable form of communication.
Infrared light pulses send information from a transmitter to a receiver. Due to the speed of light, fiber systems can provide very high bandwidth and low latencies. The fiber network is largely immune to electromagnetic interference with reliable throughput.
The fiber systems are typically an extensive network of routers and switches interconnected using fiber optic cables.
The fiber must be deployed to all automated field devices.
New technologies developed for fiber-to-the-house (FTTH) can be leveraged by utilities to reduce the cost of fiber networks. Internet providers use passive optical networks (PONs) to get fiber to homes. PONs use optical splitters (Figure 8) to direct the signals rather than having an electrically powered switch.
IP-Based Communication Systems
Most modern communication systems are IP-based. An IP network is a communication network that uses Internet Protocol (IP) to send and receive messages between devices in the field and the field and control center. An IP network is implemented in local area networks (LAN) and enterprise-wide area networks (WAN). An IP network requires all hosts or network nodes to be configured with the TCP/IP suite.
The TCP/IP suite provides end-to-end data communication by specifying how data must be packetized, addressed, transmitted, routed, and received.
Each device is assigned a unique IP address, which distinguishes it from other devices and helps initiate data communication with other devices. IP network communication occurs when a device sends a data packet to another by addressing its IP address. Similarly, the recipient identifies the sender by its IP address.
IP-based systems are interoperable, making sending messages through cellular, fiber, and radio systems to a control center possible. Virtual LANs (VLANs) should be used for DFA systems to keep the devices in a separate subnetwork.
Communication System Considerations
Feeder Automation System Selection
The utility needs to decide what automation system will best support its goals and objectives to improve its reliability key performance indicators (KPIs) and supply reliability to its customers. It could be that a combination of approaches will best meet their goals.
For example, a commercial district will likely benefit from a high-speed Category 4 system. Some commercial customers cannot tolerate short interruptions. City residential districts could benefit from a Category 3 system, and the rural districts could benefit from a Category 2 existing SCADA system. The Table on page 38 indicates the communication systems that can support different automation systems.
Leverage Existing Communication Systems
Utilities have traditionally used communication systems to support their supervisory control and data acquisition (SCADA) and AMI systems. These systems require minimal throughput and can tolerate long latencies. If the utility uses unlicensed 902-928 915 MHz radios for AMI, we can see that such radio systems cannot support a Category 5 system. If the utility use licensed 900 MHz for SCADA communications, this communication system can still not support a Category 5 system. The existing communication systems must be qualified to see if their throughput and latency will support the feeder automation system. Radios that are not IP-based might not support the requirements of the feeder automation systems.
The SCADA radio system can support Rural feeder automation. The next step will be to determine if the system can be expanded to include the numerous field devices to be automated. The systems might require additional tower assets to reach the field devices.
In summary, the existing communication system performance, reliability, availability, interoperability, deployment /expansion, and maintenance must be assessed.
Considerations in Selecting a New Communication System
If the existing communication infrastructures can support the rural feeder automation system, new communication systems must be considered for residential and commercial feeder automation systems.
Cellular: Cellular will support both residential and commercial systems. Cellular systems can provide excellent penetration and coverage in residential areas, and the latency of a cellular communication system varies based on loading. There are no tower infrastructure investments required.However, the availability of cellular communication systems can be a problem. Providers maintain equipment, and many potential interruptions must be expected. The feeder automation system must have robust features that tolerate varying latency and communication disruptions.
Licensed Radio: Licensed radio communication systems will support the residential feeder automation system. The systems provide excellent reach and penetration but will likely require investments in tower infrastructure. The low risk of interference makes the systems very reliable in maintaining performance.
Meshed radio systems can be considered but will likely not provide the throughput and latencies required by a feeder automation system. The throughput and latency of the 700 and 900MHz radios will make the feeder automation system act slower. The low risk of interference makes the systems very reliable.
CBRS Radio: CBRS radios will support both residential and commercial systems. However, the systems will likely require investments in tower infrastructure. Field device antennas might need additional elevation. The radios provide limited reach, and penetration is not as good as cellular systems. A residential area that is densely wooded could be challenging.
The CBRS radios can provide excellent performance with low latencies and high throughput. The risk of interference is lower today, making CBRS more reliable.
The CBRS systems are private and secure. The owner is responsible for maintenance.
Unlicensed Low-Frequency Radio: Unlicensed low-frequency radio communication systems will support the residential feeder automation system. However, the systems will likely require investments in tower infrastructure. That said, the radios provide excellent reach and penetration.
Meshed radio systems can be considered but will likely not provide the throughput and latencies required by a feeder automation system. Frequency-hopping radios can solve interference problems, but performance will vary based on the frequencies used.
The throughput and latency of the 900MHz radios will make the feeder automation system act slower. The risk of interference is high, making the systems unreliable in maintaining performance.
Unlicensed High-Frequency Radio: Unlicensed high-frequency radio communication (WiFi) will support a commercial FDA system. The systems will require investments in tower infrastructure. Radios provide limited reach. The penetration is not good, and it could be challenging to get radio links to all switchgear locations. Maintaining performance is difficult due to the high risk of interference. Frequency-hopping radios can solve interference problems but impact performance.
The radios can provide excellent performance with low latencies and high throughput.
Conclusion
The communication systems must support the DFA system’s data throughput and latency requirements.
Many factors can affect the successful transmission of data packets in wireless radio systems.
Over-dimensioning of the radio is required as environmental conditions can influence the throughput. In wireless systems, smaller data packets will be delivered with better reliability.
Private radio systems require infrastructure investments and maintenance.
Commercial cellular communication requires very little infrastructure investment and is readily available.
Fiber optic networks provide the most reliable communication with the best latency and throughput.
IP-based communication systems provide excellent interoperability and flexibility, simplifying integration into a Utility WAN.
Biography:

Andre Smit – is an Executive Advisor at Quanta Technology and has over 33 years of experience in protection systems for power transmission and distribution systems. He served in many different roles in the protection and control industry and has a comprehensive understating of the market. He is an industry expert in distribution feeder automation systems. He led the development of new concepts leveraging IEC61850 to automate distribution feeders using wireless communication systems. He is the principal inventor of a distribution automation system and holds 7 US and international patents in the field. He pioneered the deployment of cellular base IEC61850 Direct Transfer Trip systems for remote distributed generation sites. He has deep subject matter expertise in generator protection systems.