by Bogdan Kasztenny, Schweitzer Engineering Laboratories, Inc. Canada
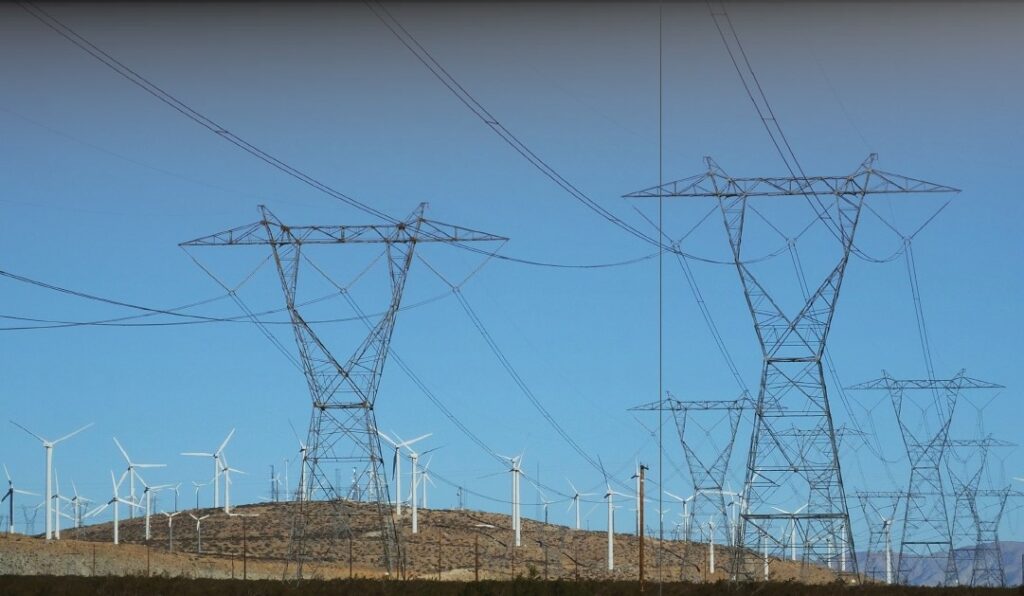
We have been fortunate in the first century of power system protection. Power grids have been remarkably stable from the point of view of failure modes and short-circuit characteristics. Components in the grid are made of conductors, insulation, and magnetic cores. Well-understood laws of physics drive both the power apparatus failure modes and the grid response to short circuits. Manufactured by General Electric or Brown Boveri, a synchronous generator outputs a negative-sequence current that is in a fixed relationship with the negative-sequence voltage. Manufactured by Siemens or Westinghouse, a power transformer draws an inrush current that is rich in odd harmonics. Currents measured around a healthy bus follow Kirchhoff’s current law and add up to zero, irrespective of the make and model of the circuit breakers around that bus.
A voltage dropacross a transmission line follows the line current and the line impedance, irrespective of the style of towers and type of conductors and isolators. It is true that equipment manufacturers have competed, and designs have improved. However, using better magnetic steel to reduce generator losses or using synthetic insulation in place of paper and oil has not altered the fundamental characteristics of the power grid.
These characteristics have been persistent, and they have allowed development of power system protection principles as we know them today. The overcurrent, distance, directional comparison, and differential protection principles are rooted in the laws of physics, but they also rely on the characteristics of the grid. Over the years, relay designers have continued to discover more subtle fault characteristics and have used them to enhance protection performance. We noticed that the phase angle between the zero-sequence and negative-sequence currents is a reliable indicator of the fault type and started using it in faulted-phase selection logic. We noticed that the phase angle of the negative-sequence current at the relay location is a good indicator of the voltage phase angle at the fault location during resistive faults and started using it to polarize the reactance distance elements.
We noticed that the pre-fault voltage represents the phase angle of the source that drives the fault current and started using memory voltage to polarize the mho distance elements. The list goes on.
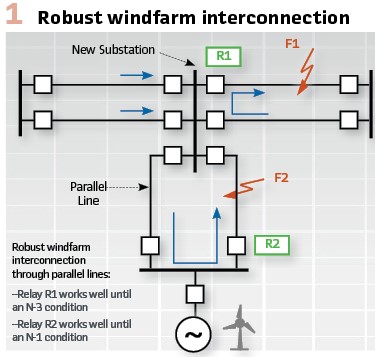
Driven by the need to innovate and compete, protective relay designers have extracted major and minor characteristics of the grid and used them in protective relays. The extraordinary persistence of these characteristics, combined with progress in relay technology, encouraged the process. Static relays allowed more design freedom than electromechanical relays. Microprocessor-based relays opened the flood gates for protection logic that responds in sophisticated ways to subtle relationships between the relay inputs. As a result, many protection principles that we use today are closely coupled with the short-circuit characteristics of the grid. Only principles that are based on the most basic laws, such as the differential principle, remain relatively universal and apply to any electrical circuit.
As power system protection became a mature field, the industry’s attention shifted from discovering new principles to providing additional functionality (fault recording and locating, for example), improving protection channels and making them more economical, improving ease of use, reducing lifecycle cost, and so on. We took the persistent characteristics of the grid for granted. Today, we do not discover new protection principles but expect to learn them from textbooks. We have shifted our attention to cost and ease of use, we pursue ideas of digitization, and branch into indispensable – but nonetheless adjacent – spaces of time synchronization, communications and networking, and software. A brief analysis of working group titles in key technical organizations reveals where our focus has shifted.
The Disruption
Over the years, series compensation, high-voltage direct-current (HVDC) links, static VAR compensators, and phase-shifting transformers were warning signs of how power systems may change. Historically, the volume of these special applications has been low, and equipment manufacturers played an important role in solving protection problems they created. Two decades ago, however, the progress in power electronics and material science, especially in applications to solar cells and wind turbines, combined with the shift in societal attitudes toward global warming and renewable power, fueled the rise of wind and solar power generation. The emergence of wind and solar power is the story of innovation and disruption. Initially underperforming in terms of cost and scale, these new technologies matured and scaled up. Although no longer subsidized and politically driven, the new sources are being installed at an increasing pace, dramatically changing the grid. The intermittent nature of the renewable power invites development and deployment of energy storage devices. Developments in power electronics and undersea cable technology have increased the number and size of HVDC links. Substantial price differences in a deregulated market create financial incentives for large power transfers across long distances by using HVDC links.
A wind generator, a solar farm, an HVDC link, and a large battery storage site all may respond differently to system faults. Their short-circuit responses are universally different than that of a synchronous generator, but also, they differ among themselves. Even though limited by the laws of physics, the short-circuit response of new sources is governed by their control algorithms. Therefore, the short-circuit response can change when these algorithms change because of a firmware update or when factory setpoints change. In the early days of wind and solar power, protection engineers learned the hard way that the fault response of these new sources is different and quickly labeled them as unconventional sources. Initially, these sources had a relatively low power rating compared with the ratings of the nearby synchronous generators. This allowed their interconnection, but not without hiccups and the uneasiness of protection engineers who had to guarantee system protection performance under technical uncertainty and societal pressure to proceed.
The new sources disrupted the protection engineering field. Some practitioners tried to “wish the problem away” by demanding a fault response identical to that of a synchronous generator. Others put their chips on modeling. The wall between the silos of protection engineering and power electronics did not help. The source manufacturers moved relatively fast, motivated by opportunities and profits. Naturally, the manufacturers and engineering companies downplayed the technical issues when pursuing customers and contracts. The utility protection engineers, relay manufacturers, and industry organization played, and continue to play, catch-up.
So far, protection engineers have stayed away from imposing any protection requirements on the new sources. The historically stable nature of the power grid brought about over-confidence: we were able to figure things out in the past, therefore we will solve all new problems too. When the idea of mandating the fault response of unconventional sources was first discussed some 15 years ago, it was met with fervid opposition – “we, protection engineers, do not get to dictate how the primary equipment is built.” It is true that we have not done so in the past, but that is because we have not needed to.
As with many new and dynamic fields, considerable hours of research and development have been dedicated to unconventional sources, especially those that include inverters and associated control algorithms. Over the years, these control algorithms have been refined, and today they accommodate fault ride-through and many other grid requirements. New ideas are proposed in the realm of frequency response, synthetic inertia, reactive power response, and so on. And of course, the industry is driven to make the new sources “better, larger, cheaper,” which will continue to fuel their proliferation but also bring continuing change in the source characteristics. While protection engineers apply self-restraint, other power system disciplines keep proposing and mandating characteristics for the new sources.
A Technical Problem or a Cost Challenge?
Renewable power started as an activity subsidized by taxpayers and ratepayers. Tremendous pressure was applied to make the wind and solar sources economically viable. Initial drafts of utility interconnection standards were often softened to avoid technical solutions that were deemed cost prohibitive. The challenges we face today are technical problems as much as economical constraints.
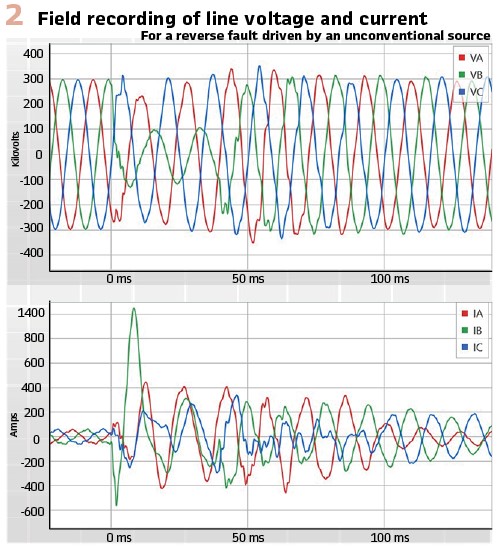
The issue of bidirectional power flow when adding distributed generation to medium voltage distribution feeders is an excellent example of the dilemma of cost vs. technical challenge. Bidirectional power flow is nothing new to protection engineers. We have protected transmission lines in the interconnected grid for the last 100 years by using a variety of standard solutions. It is only the assumption that the job must be done with time-coordinated overcurrent relays that creates the challenge. The challenge is not new but arises from the imposed cost constraints and the inability to switch to unit protection schemes (directional comparison or differential). This dilemma between cost constraints and genuine technical challenges is more subtle at the transmission level when interconnecting large unconventional sources, but the principle remains the same: some technical challenges arise from not investing more in the affected area of the grid before interconnecting unconventional sources. The focus is instead on reaping benefits from the past investment in the grid.
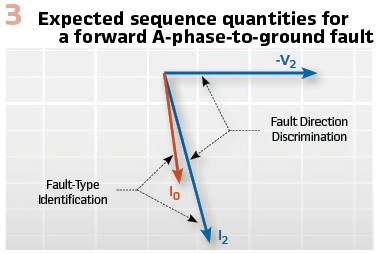
It is important to realize that short-circuit protection problems are mostly limited to line protection. Power transformers, buses, and capacitor banks use unit protection and benefit from the strength of the differential and unbalance protection principles. Challenges in line protection occur when an unconventional source dominates the short-circuit voltage and current at the line terminal. Unless and until the unconventional sources become a dominating type of power in the interconnected grid, we can count on fault current contributions from synchronous generators to alleviate the line protection problems. From this perspective, we have a range of tools to address, or to at least mitigate, the problem. Consider the following solutions:
- Using line current differential (87L) schemes. The 87L schemes operate on the total fault current from all line terminals. Current contribution from synchronous generators dominates in the 87L operating signal and ensures protection dependability and selectivity of single-pole tripping
- Using breaker failure protection with direct transfer trip and redundancy of protection systems, communications channels, control power, and breaker trip coils, to eliminate reliance on far-reaching distance elements for backup system protection
- Interfacing the unconventional source in such a way that multiple lines connect it to the bulk electric power system through diverse paths (for example, by inserting a substation into two parallel lines, see Figure 1). By doing so, the unconventional source becomes a sole source of the line fault current only under N-2 or N-3 conditions
- Avoiding compounding protection challenges, such as interfacing an unconventional source by connecting it to a series-compensated line with a large degree of compensation and then splitting the old line into new sections, making the new line overcompensated
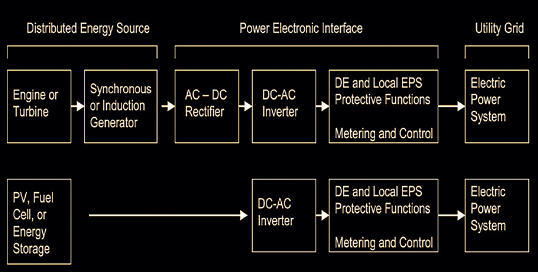
Every technical problem has cost constraints. However, in the case of integrating unconventional sources, these constraints are not only significant but are also often overlooked. A significant portion of academic research in this field is misdirected and fails to produce valuable output because researchers do not realize that their solutions have costs and if these costs were acceptable, simpler solutions would already be available to us.
Line Protection Challenges
The unconventional sources do not supply large currents during system fault conditions (see Figure 2). Therefore, they cannot maintain voltage at the line terminal, and as a result, we think of them as weak sources. Additionally, the positive-sequence current of the source does not typically follow the inductive phase angle relative to the voltage. The source’s low inertia allows the positive-sequence voltage angle to drift relatively quickly during fault conditions. Most importantly, the response of these sources to the negative-sequence voltage is not a negative-sequence current that reflects a fixed negative-sequence impedance, but a current that is aggressively modulated with a phase angle that varies with time. This behavior results from adopting the variable frequency drive (VFD) methodology in the inverter-based source designs. The VFD methodology was the tried-and-true approach, but it resulted in a solution that effectively squelches the phase imbalance when that imbalance occurs, such as during a fault in the grid. As a result, we face the following fundamental line protection challenges:
- The negative-sequence directional elements do not work reliably. If distance elements use the negative-sequence directional supervision, distance protection issues will arise
- Current-based faulted-phase selection logic does not work well because of the nature of the fault current contribution. This problem boils down to the behavior of the angle between the negative-sequence current from the source and the zero-sequence current from the interconnection transformer. Unreliable fault-type identification creates issues for distance elements and diminishes selectivity of single-pole tripping
- Distance elements face issues with their polarizing signals. Low inertia of the unconventional sources creates issues for the polarizing voltage of the mho elements, especially when using memory polarization. Unreliable negative-sequence current creates issues for polarizing the quadrilateral elements
- Without reliable distance elements, terminals with unconventional sources cannot provide remote backup (stepped distance protection) for the system, and instead, local backup is required (breaker failure protection, transfer tripping, and redundancy)
The Quest for Modeling
Faced with the uncertainty of the fault response, protection practitioners embarked on the quest to develop procedures for obtaining digital models of the new sources. Industry working groups have been started. Contracts have been written to guarantee availability of the models in time for engineering of the interconnection. Performance-based contracts with timelines and built-in penalties have been deployed to guarantee the models would continually be refined based on in-service data. The wind and solar manufacturers pushed back, citing the proprietary nature of their designs and intellectual property rights. Black-box methodologies for sharing models have been considered.
Although the models were important for the dynamic system analysis and overall understanding of the issues, they were not that useful for utility protective relay engineers. An accurate model of a source with characteristics that challenge available protection methods does not solve the issues with protection; it only reveals them. Setting today’s relays by using such a model to fine-tune settings and write programmable logic is risky because the models have limited accuracy. Moreover, the source response may change overnight as a result of a firmware update or changes to factory setpoints, rendering the model obsolete. Updating relay settings and recommissioning relays after a firmware update in the unconventional source controllers is not a sustainable engineering method. The push for modeling reflects our collective experience in the last 100 years – the object of protection is static; once we discover its properties, we can protect it. This approach worked for iron and copper apparatuses, but it is not applicable to grids with power sources that incorporate power electronics and digital signal processors running arbitrary and proprietary control loops.
Early Solutions
In the absence of solid engineering rules for protecting lines in the vicinity of unconventional sources, and because of the uncertain fault response of these sources, relay practitioners solved the problem by turning to the one persistent characteristic of the source that they could count on: namely, its low power rating.
Weak-Infeed Logic: Line terminals with unconventional sources are treated as weak terminals. Enabling a weak-infeed echo and trip logic provides adequate line protection. Using negative-sequence directional elements is avoided because they may assert in the reverse direction for line faults. If single-pole tripping is applied, the phase selection logic may need to be modified to use voltages instead of, or in addition to, currents.
87L Schemes: When a suitable digital protection channel is available, an 87L scheme is a good solution for line protection. A strong fault current contribution from the system terminal(s) allows dependable protection with the security and phase selectivity that is inherent in a differential scheme.
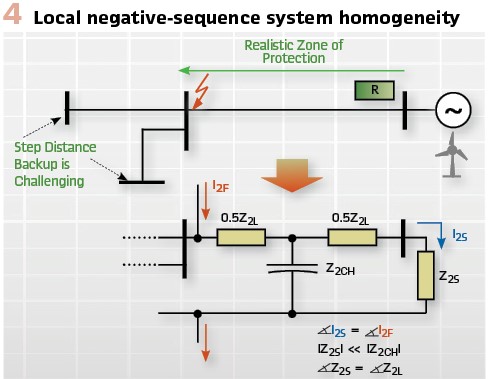
The weak-infeed logic and the 87L schemes will continue to work adequately as long as there is sufficient fault current contribution from traditional generators. However, both these schemes are based on communications and require backup should the channel fail. Distance elements do not work reliably with currents from unconventional sources, taking the stepped distance schemes off the table. Redundant and diverse channels, including direct transfer tripping from the strong terminal(s), time-coordinated ground direction overcurrent protection, and undervoltage tripping at the weak terminal are, for now, acceptable backup solutions for line faults during protection channel failures.
The Crucial Role of the Negative-sequence Response
Addressing issues with the negative-sequence response would solve many line protection challenges. Negative-sequence current that follows the negative-sequence voltage would enable reliable fault direction detection. Negative-sequence current that follows the negative-sequence voltage would also follow the angle of the zero-sequence current and therefore would enable correct fault-type identification. A negative-sequence current angle that represents the angle of the fault current in the surrounding system would allow proper polarization of reactance characteristics and therefore would allow distance protection applications, at least for unbalanced faults. Solving the directional, phase selection, and distance polarizing issues would go a long way toward improving line protection in applications near unconventional sources.
Line protection operating conditions would considerably improve if the negative-sequence current met the following characteristics (see Figure 3):
1. The negative-sequence current must lag the negative-sequence voltage by about 85 degrees (a value typical for synchronous generators and power lines); it is preferable if the angle stabilizes within about one power cycle
2. The negative-sequence current magnitude must be sufficiently high compared with the positive-sequence current to allow local relays to measure it reliably; 10 to 20 percent is sufficient
3. The negative-sequence source impedance that results from outputting the negative-sequence current must be low enough to keep the negative-sequence network homogeneous and by doing so, keep the angle of the local negative-sequence current close to the angle of the fault current for faults in the surrounding system (see Figure 4). At a minimum, the negative-sequence impedance (Z2S) shall be a few times lower than the shunt (charging current) reactance of the line (Z2CH)
Characteristics 1 and 2 are the easiest to achieve and they allow local directional detection and fault-type identification. Characteristic 3 is more difficult because it requires the source to output relatively high negative-sequence current. However, it is more powerful than Characteristics 1 and 2 alone because it allows application of the quadrilateral distance elements. A good compromise is to require only as much negative-sequence current as is needed to keep the system homogeneous for faults at the remote line terminal(s). This would enable distance element applications at the terminals with the unconventional sources for line faults (including backup for the remote bus), while dispensing with remote backup applications for stations that are two or more buses away.
Transient-Based Line Protection
The last several years saw a rebirth of transient-based line protection based on incremental quantities and traveling waves. This approach to protecting lines in the vicinity of unconventional sources is viable because it leverages the characteristics of the line, not the source. The energy stored in the line capacitance and inductance fuels the traveling waves (see Figure 5). These high-frequency signals follow the line characteristics irrespective of the power source. The transient-based methods use the playbook of the first 100 years of protection – they leverage discoverable and persistent characteristics of the protected apparatus. Now, however, instead of using the power frequency signals, the principles use signals in the high-frequency spectrum. This time-domain approach to line protection has been made possible by advancements in signal processing (megahertz sampling) and communications (direct fiber protection channels). The first relays of this kind were deployed in 2017 and have an excellent track record in the field. Based on transients, however, these new line protection principles can only operate within a time window of a few milliseconds. Once the transients die out and the operating window closes, the time-domain protection will not operate; it requires backup protection. The backup protection must be based on power frequency signals, and as such, it is dependent on and affected by the sources.
The Road Forward
Transient-based methods will play a major part in line protection, especially when the penetration of unconventional sources increases, the fault contribution of synchronous generators declines, and the transient stability requirements call for ultra-high-speed relay operation. The transient-based protection methods require phasor-based backup, however.
The lack of persistent characteristics in the unconventional sources prevents innovation and development of new phasor-based protection methods. Relay manufacturers cannot be expected to release new protection elements for unconventional sources as long as these sources have fluid characteristics that can change overnight with a firmware update or from one generation to the next because of advancements in the underlying technology.
Identifying and mandating – through grid codes – persistent source characteristics during fault conditions must be our long-term solution. Persistent source characteristics would unleash progress and innovation by creating a clear demarcation point, as follows:
- Source manufacturers would have a specification to work toward
- Users could verify the source performance and confirm compliance
Relay manufacturers would have a persistent fault characteristic to work with when modifying their line protective relays, especially in the realm of directional elements, fault-type identification, and distance polarizing methods
In the first 100 years of protective relaying, we did not need a standard to tell sources in the grid how to respond to faults. The sources responded in a predicable way on their own. All we needed to do was discover that response. The new sources of today need to have their response standardized to bring about persistent characteristics.
The laws of physics will put limits on what the standardized response of a source can be. An inverter-based source cannot respond exactly like a synchronous generator, but also it does not have to. The future standards should also be sensitive to cost and reliability. Protective relays can measure subtle features in input voltages and currents. Therefore, the sources do not need to output very high currents to facilitate reliable protection. It is not necessarily the current level that matters, but how the current is controlled.
An organized and methodical effort is needed to identify and codify the source characteristics that would enable reliable system protection. This work must be a multidisciplinary effort involving not only the new source experts and protection engineers, but also other disciplines that have already forced grid codes to solve their own challenges, such as limiting overvoltage conditions. Various industry bodies have an important role to play in organizing and supporting this effort. Our working group titles must drop phrases like “protection issues” and embrace solutions. We need a standard titled “Fault Response Requirements for Unconventional Sources.” Most importantly, utilities must play a key role in enforcing the new grid code.
It is time to put the excitement of the early days of discovering new technology behind us and start following a predictable process of standard engineering. Standard interfaces and demarcation points support innovation and progress; they do not hinder it. In the case of unconventional generation, the precious standard interface and demarcation point is a persistent fault characteristic of the new sources. The sources will no longer be nonstandard if they have persistent and standardized characteristics. The source and relay manufacturers would continue innovating, while the users would be able to engineer systems following standard practices.
Other changes will be needed as well, such as pivoting away from remote backup through stepped distance schemes and relying more on breaker failure protection, direct transfer tripping, and redundancy. Synchronous generators are strong beacons that force large signal changes and allow relays in a relatively large area to see a fault. The new sources cannot play that role. In the new grid with a large penetration of unconventional sources, protection will have to be more localized and will have to rely on protection channels rather than short-circuit current paths for gaining system-wide information.
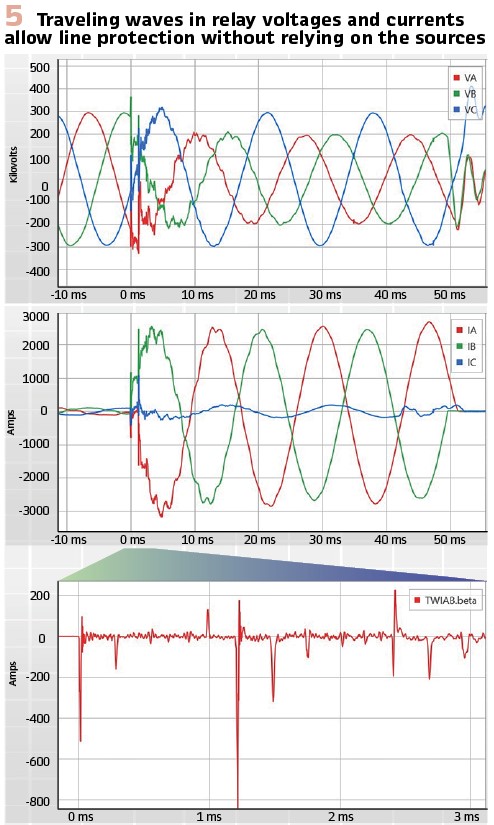
Biography:

Bogdan Kasztenny specializes in power system protection and control. In his decade-long academic career, Dr. Kasztenny taught power system and signal processing courses at several universities and conducted applied research for several relay manufacturers. Since joining the industry in 1999, Bogdan has designed, applied, and supported protection, control, and fault-locating products with their global installed base counted in thousands of installations. Bogdan is an IEEE Fellow, a Senior Fulbright Fellow, a Distinguished Member of CIGRE, and a registered professional engineer in the province of Ontario. Bogdan has authored over 220 technical papers and holds over 55 patents.