Can AMI Systems Become More Grid-centric AGI Systems to Support Challenges on the Distribution Grid?
by David G. Hart and Julio Romero Aguero, Quanta Technology, USA, and Aleksi Paaso LUMA Energy, Puerto Rico
Challenges on the distribution grid are driving the need for new solutions at the substation, feeder, and application levels. All utilities are adapting to (i) the growing adoption of Distributed Energy Resources (DER), (ii) expanding electrification, and (iii) the increasing frequency and severity of weather events that are impacting the distribution grid and driving the need for resiliency improvement.
To address customer expectations regarding service reliability, resilience, and power quality, utilities are implementing grid modernization plans. One of the key enablers for these plans is the availability of communications across the distribution grid. When Advanced Metering Infrastructure (AMI) was first introduced, one of the goals was to provide last mile communications capabilities to the grid. While initial AMI deployments have greatly advanced metering capabilities, outage detection, and other functions, new AMI systems have the potential to be leveraged for more functions to support grid modernization plans. This article discusses key aspects of grid modernization plans, and describes three components, Feeder of the Future (FoF), Substation of the Future (SoF), and AMI, in more detail.
Grid Modernization
Traditionally, a utility’s primary concerns were the delivery of safe, affordable, accessible, and reliable power to its customers. While these remain critical aspects, the utility landscape is changing due to technical and societal drivers, and new features have emerged and grown in importance, including sustainability, flexibility, security, and resilience. This is conceptually illustrated in Figure 1.
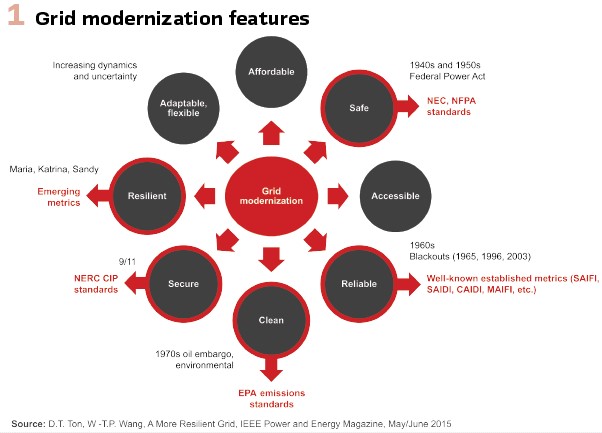
Grid modernization allows preparing the power delivery grid to address these drivers, which include:
- Aging infrastructure
- Stresses and requirements imposed on the existing grid by the adoption of new technologies, such as DER and electrification
- Evolving changes to climate and weather patterns (e.g., more frequent and more severe storms and catastrophic events, such as hurricanes and wildfires)
- Impacts from human events (e.g., sabotage, terrorism, etc.)
- Evolving expectations of customers regarding reliability, resilience, and power quality
Grid modernization enables key capabilities and features for a modern and future grid, including :
- Improved reliability for everyday operations
- Increased sustainability through energy-efficiency, demand response, and renewable resources
- Greater resiliency to hazards of all types
- Enhanced physical and cyber security from an increasing and evolving number of threats
- Long-term affordability to maintain economic prosperity
- Superior flexibility to respond to the variability and uncertainty of conditions at one or more timescales, including a range of energy futures
The evolution of the distribution grid is illustrated in Figure 2.
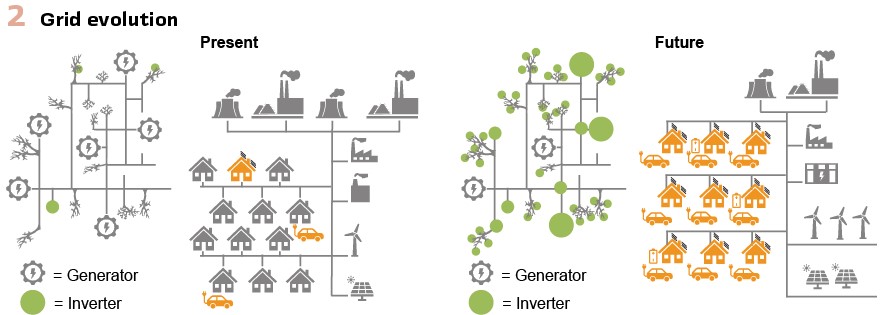
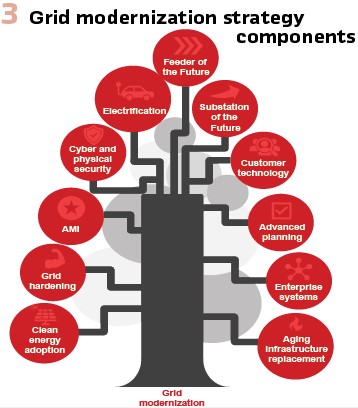
A grid modernization roadmap leverages the industry’s best practices and considers emerging technologies to set the basis for transforming and preparing for the future according to the utility’s vision and goals. The roadmap outlines key initiatives or programs needed for the organization to improve the overall performance of its power delivery system. Examples of grid modernization strategy components are shown in Figure 3.
A grid modernization roadmap consists of a prioritized portfolio of programs and corresponding investments. Programs are projects or initiatives that can be implemented to realize the desired functionality, capability, or other specific utility goals. For example, if one of the utility’s key drivers is reliability improvement, then programs that reduce the frequency and duration of service interruptions are potential options to be considered (e.g., implementing feeder automation, deploying an outage management system (OMS), or enhancing vegetation management practices). If a utility wants to support providing enhanced information to customers, program options could include website upgrades to enhance customer interaction or an interface to hourly consumption from an AMI program with smart meters.
For the distribution grid, communications are typically available for the substation (e.g., ability to monitor and control circuit breakers) and may be available at selected locations along distribution feeders (e.g., reclosers, switches) but last mile communications along the feeder (e.g., service transformer level) and at customer premises are often missing.
The Feeder of the Future (FoF)
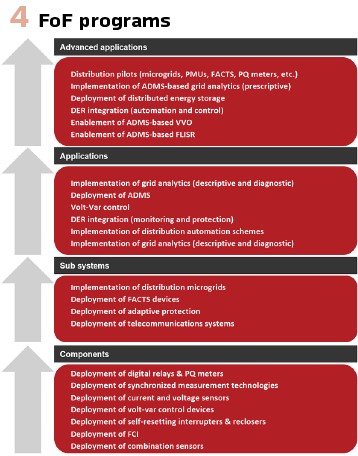
The FoF component of a grid modernization plan addresses key technology areas of interest to utilities, including feeder monitoring, protection, automation, and control, DER integration, microgrids, telecommunications, Advanced Distribution Management Systems (ADMS), grid analytics, and pilot projects, which are conceptually shown in Figure 5. Objectives of the FoF component include modernizing the power delivery infrastructure to 1) prepare or “future proof” the distribution grid, to a reasonable extent, for the adoption of DER (e.g., distributed generation, distributed energy storage, etc.), electrification and emerging technologies, and 2) enable the required capabilities to meet the utility’s strategic objectives and regulatory requirements, such as reliability and resilience improvement (e.g. harden the grid against major weather events), decarbonization (e.g., adoption of DER and electric vehicles), and climate change preparedness.
Some of these areas involve the implementation of mature technologies, such as distribution automation, while others address emerging areas, such as synchronized measurements. For mature technologies, direct deployment is suitable, while for emerging technologies, the recommended approach is first exploring the concept in a pilot setting that allows investigating a variety of scenarios and develop guidelines and processes, and then operationalize the technology and conduct a wider-scale deployment.
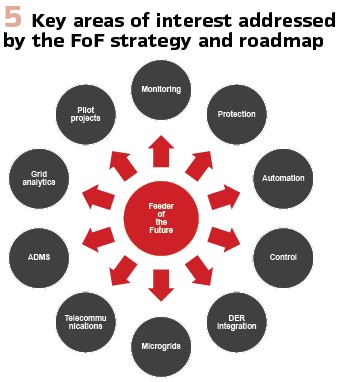
Examples of FoF programs are outlined in Figure 4. In prioritizing these programs, it is important to consider the maturity of the specific technologies, relative implementation complexity, importance and urgency of the need that the component is expected to address, interdependencies with other components, and a component’s potential to help achieve strategic goals. Here it is worth noting that all components are important, particularly in the long term, while some are more important in the short term.
The implementation schedule of the FoF component is typically divided in timelines such as short term (zero to five years), mid-term (five to 10 years), and long term (10 to 15 years). The proposed implementation timeline is intended to balance 1) the urgency to address critical short-term distribution system needs, such as reliability improvement, 2) the need to modernize the grid to comply with industry accepted practices and prepare it for the significant changes introduced by DER adoption and climate change, and 3) the level of effort involved in the implementation of the selected components.
While it is difficult to “future proof” the distribution grid given the uncertainties and risks associated with transformational and disruptive technologies, clean energy adoption, the impact of severe weather events, need to balance costs, etc., the FoF concept provides a strong foundation and proactive approach that will ensure a utility is ready to prevent or address expected challenges. It is important to highlight that the intention of the roadmap is not to “goldplate” the grid, but to proactively identify investments that will be needed as the grid evolves, and to schedule their implementation accordingly.
Substation of the Future (SoF)
Another key component of a grid modernization plan is the Substation of the Future (SoF). There is an urgent need for substation modernization given the increasing importance of the interaction effects between transmission and distribution systems, such as reverse power flow through substation transformers as well as voltage fluctuations and protection coordination challenges caused by DER proliferation. Additionally, transportation electrification integration is creating a growing need to monitor substation assets in real-time to understand how charging infrastructure impacts asset loading (e.g., substation transformers), power quality, and equipment operation (e.g., load-tap changers). Similarly, complying with energy storage mandates and targets require advanced substation monitoring, automation, and control capabilities.
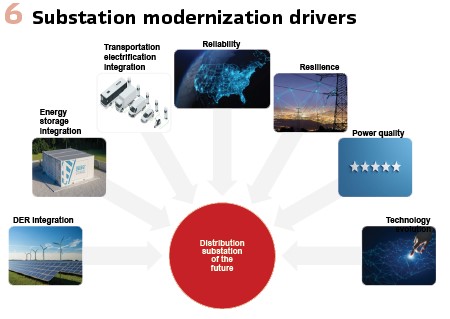
Additional drivers for substation modernization are shown in Figure 6 and include reliability, resilience, and power quality improvement. Technology evolution, such as the proliferation of intelligent electronic devices (IED), including microprocessor-based relays and power quality meters, can enable key new capabilities to plan and operate modern power delivery systems that were not available in traditional equipment (e.g., electromechanical relays). This can also allow implementing new concepts, such as substation-level microgrids and Virtual Power Plants (VPP) and intentionally islanding.
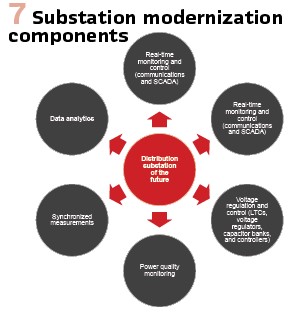
SoF entails the deployment of key monitoring, protection, automation, and control technologies along with data analytics solutions to replace existing equipment and enable new capabilities and functionalities. SoF technologies include:
1. Real-time monitoring and control: This includes the deployment of sensors and communications systems and SCADA to collect and transmit key substation data in real time to control centers (e.g., voltages, currents, power flows, equipment status, temperature, etc.) and enable the implementation of real-time operation of the distribution grid and advanced analytics.
2.Advanced protection, automation, and control: This comprises the deployment of modern microprocessor-based relays with advanced functionalities to replace electromechanical relay technology (e.g., to provide the ability to implement adaptive protection settings). This also includes ensuring that suitable protection functions are in place for reliable and safe operation under bidirectional power flow conditions through distribution feeders and substation transformers. This component also consists of implementing the IEC 61850 communications standard to enhance protection, automation, and control capabilities and enable further integration of DER and electric transportation while maintaining or improving reliability performance.
3. Voltage regulation and control: This includes the deployment of voltage regulation and control equipment (load tap changers, voltage regulators, capacitor banks, and respective controllers) with the ability to regulate and control voltage under bidirectional power flow conditions and fluctuating power flows. This includes the integration or coordination of these devices with the Volt-Var Optimization (VVO) implementation envisioned by the FoF initiative.
4. Power quality monitoring: This includes the deployment of power quality meters to monitor and evaluate electric power waveforms at substations and key distribution feeder locations (e.g., sensitive customer sites) and proactively assess and identify solutions to prevent or mitigate DER impacts (e.g., voltage fluctuations, harmonics, etc.)
5. Synchronized measurement technology: This includes the deployment of phasor measurement units (PMUs) to collect high-resolution time-synchronized data (magnitude and phase angles of voltages and currents), improve wide-area situational awareness of the power delivery system and DERs, and understand and improve overall system performance.
6. Data analytics: This includes the collection, processing and analysis of real-time data provided by Intelligent Electronic Devices (IED) such as digital relays, power quality meters, PMUs, and sensors for substation and wide-area situational awareness and implementation of other applications such as asset condition assessment and predictive analytics.
SoF will increase real-time awareness of the overall power delivery system and help understand, prevent, and mitigate DER and transportation electrification impacts. Substation modernization can enable the further adoption of DER, battery energy storage systems, and transportation electrification; improve reliability, power quality, and resilience of the power delivery system; and enhance asset health, maintenance, and overall safety.
Overview of Advanced Metering Infrastructure (AMI)
An AMI program implements a two-way remote meter reading capability that includes the smart meters, field area network (FAN) communications to the meters and other grid-edge devices, a wide area network (WAN) to the system access points, and the system head-end applications that interface to systems such as billing, outage management, etc. (Figure 8 presents a functional overview of an AMI system.) Smart meters have functionality such as load, demand, and voltage profiling that was previously only available for large commercial and industrial (C&I) customers but is now available at every metering point. With two-way communications, an AMI system can provide additional information such as more granular consumption data and power quality information, as well as outage notification, water leak detection, and remote connect/disconnect.
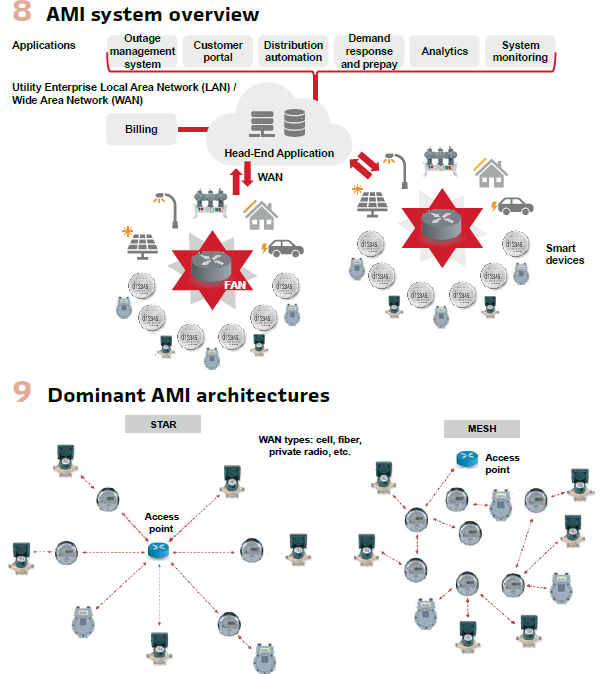
There are many different communications architectures in AMI systems, and while these include some very expensive implementations like “fiber-to-the-meter”, the two dominant architectures typically utilized are the “star” (a.k.a. point-to-multipoint) and “mesh” (a.k.a. peer-to-peer) architectures (see Figure 9).
The star implementation generally has multiple elevated base stations that communicate directly to field devices. In some cases, battery-powered devices such as gas and water meters communicate through an adjacent electric meter, but direct communications to and from these devices and the base station are usually possible. In a mesh architecture, electric meters register to the access points – either directly if they have a strong enough radio frequency (RF) signal or through a peer electric meter that has a good signal. These systems usually require that battery-powered devices communicate through an electric meter, or a repeater installed specifically for this purpose.
There are advantages to each type of architecture. For dense deployments where the electric, gas, and water service areas almost completely overlap, mesh implementations can lead to lower deployment costs since there might be little-to-no network-specific equipment other than meters. For low-density deployments or deployments with significant gas and/or water-only service territory, the star approach can be more attractive as it tends to be simpler to implement and operate.
Of course, these are very general distinctions, and many other considerations can influence architecture selection. Ultimately, the physical constraints, network maintenance, available functionality, and desired functionality must be considered when selecting an AMI system.
AGI: AMI as a Foundational Component of Grid Modernization
AMI has greatly improved the quantity and value of information provided by meters. In addition to electrical revenue metering to support the meter-to-cash process, modern smart meters provide additional information such as bi-directional metering needed for residential renewables, interval energy consumption to customer portals to allow consumer monitoring, prepay options to reduce barriers to electric service, and voltage monitoring for system analysis. In case utilities provide water and electricity, AMI-equipped water meters enable remote meter reading, but also provide leak detection to consumers and loss analysis to utilities.
To meet the new challenges, the industry and AMI providers need to the focus of AMI systems from a metering centric focus to the meter as one component of an Advanced Grid Infrastructure (AGI) that addresses the needs of a modern grid as highlighted above.
While AMI systems have provided a major step forward over earlier manual or drive by meter read processes, AMI is typically not fully utilized for additional applications as part of a grid modernization plan. For example, AMI can be utilized for:
- Feeder automation including communications to reclosers, switches, fault current indicators (FCIs), capacitor banks, etc.
- Demand response systems
- Smart streetlights
- Validation of the distribution model including mapping of meters to transformers, transformers to phases to support re-configuration schemes, load balancing, or planning
- Transformer overload monitoring due to EVs
- PEV or EV detection and/or submetering
- DER interface to ADMS or DERMS
- Water loss detection.
The new functionality of the AMI system to support validation of the distribution model enables improved system monitoring and analytics. Some of the applications typically implemented today are shown in the lower half of the two figures below. By taking a more holistic approach, AMI can support additional functions shown in half of the figures above. In practice, this means that for an AGI solution, distribution automation system requirements must be as carefully understood as the metering requirements. Advanced applications involving communications to EV or DER at the residential level need to be specified as part of the overall system rollout to help realize the FoF or SoF goals. (Figures 10, and 11).
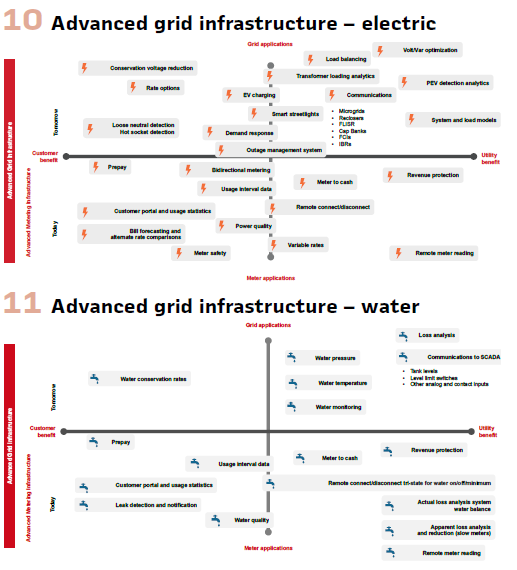
Conclusion: As utilities continue to drive decarbonization initiatives, integration of renewables and DER, adoption of electric transportation, and climate change readiness, there will be an increasing need to understand and proactively manage distribution system assets to optimize operations, improve performance, and minimize (or prevent, to the extent possible) system disruptions. Given the impacts on the distribution grid, it is important to include the SoF and FoF concepts as part of an overall grid modernization plan. The SoF concept enables advanced real-time monitoring, protection, automation and control capabilities, and therefore, depends on a reliable communications infrastructure. The FoF concept involves the deployment of feeder devices, subsystems, and applications. Communications continues to be a major issue for the distribution grid to implement many of these features. To achieve communications across the distribution grid to connect the various components and enabling new applications, it is important to examine the use of the AMI network as a cost-effective solution. Working with utilities, AMI providers can make this a reality and shift the focus from metering centric to grid centric, i.e., transitioning from AMI 2.0 to AGI 1.0.
Biographies:

David Hart – PhD, Executive Advisor, Vice President, Protection, Control & Automation, Quanta Technology, LLC has more than 30 years of experience in the power industry, including protection and control, power system automation, smart metering, and AMI systems. Before his work at Quanta Technology, David held management positions at ABB, where he developed protection products and later developed AMI products and systems at Elster, where he was responsible for the engineering, product management, and quality organizations. At Quanta Technology, David and his team provide consulting support to utilities on protection and control, AMI systems, feeder automation, analytics, coordination studies, and advanced applications. As the direct head of Protection, Control, and Automation area, he guides the overall business strategy, client and program proposals, and project execution. David holds a PhD in Electrical Engineering from Clemson University and is a senior member of IEEE/PES. His work has earned significant recognition throughout his career, including more than 30 technical patents and numerous articles in industry publications.

Julio Romero Aguero – PhD, MBA, Executive Advisor, Vice President, Strategy & Business Innovation, Quanta Technology, LLC has 27 years of experience, and provides leadership to Quanta Technology in many different areas. He has developed solutions in these areas for electric utilities and regulatory boards in the USA, Canada, Latin America, the Caribbean, and Asia. He is a Senior Member of the IEEE and currently serves as Vice President, Membership & Image in the IEEE PES Governing Board, served as Chair of the 2020 and 2021 Innovative Smart Grid Technologies Conference – North America (ISGT – NA), Chair of the IEEE Distribution Subcommittee, Chair of the IEEE Working Group on Distributed Resources Integration, Editor of IEEE Transactions on Power Delivery, and Editor of IEEE Transactions on Smart Grid. He is a Distinguished Lecturer of IEEE PES and an Adjunct Professor at University of North Carolina at Charlotte.

Dr. Aleksi Paaso – Vice President of Distribution Engineering and Investment Strategy, LUMA Energy. Previously Vice President of Grid Modernization with Quanta Technology, Aleksi has also held various utility leadership and technical roles at ComEd/Exelon leading the company’s reliability, resiliency, emerging technologies, and planning functions. He also led the initiative of building the first cluster microgrid in the U.S. and the largest grid energy efficiency: voltage optimization. Aleksi has served as expert witness in several regulatory proceedings. He holds a PhD in Electrical Engineering from the University of Kentucky and is the current Chair of the IEEE Power & Energy Society’s Industry Technical Support Leadership Committee.