Grid innovations and digital transformation at power substations are accelerating the energy transition for global utilities
By Mital Kanabar, GE Grid Solutions – Grid Automation, and Claudia Cosoreanu, Global PM & CTO, Canada
Key Drivers in the energy transition: Energy industry is undergoing significant transition from fossil fuel to sustainable energy sources driven by decarbonization, as well as UN’s Sustainable Development Goals (SDG) such as, climate action, affordable and clean energy initiatives. This transition needs to balance energy trilemma problem among energy security (incl. reliability and resilience), affordability or equity, and environmental sustainability. The energy transition is at hand, but its success relies on our ability to adapt our aging power grid infrastructure to support the entire ecosystem around it, as illustrated in the Figure below. Innovation and the digital transformation of the grid substation are crucial to evolving our technology rapidly enough to meet the world’s rising demands of green energy.
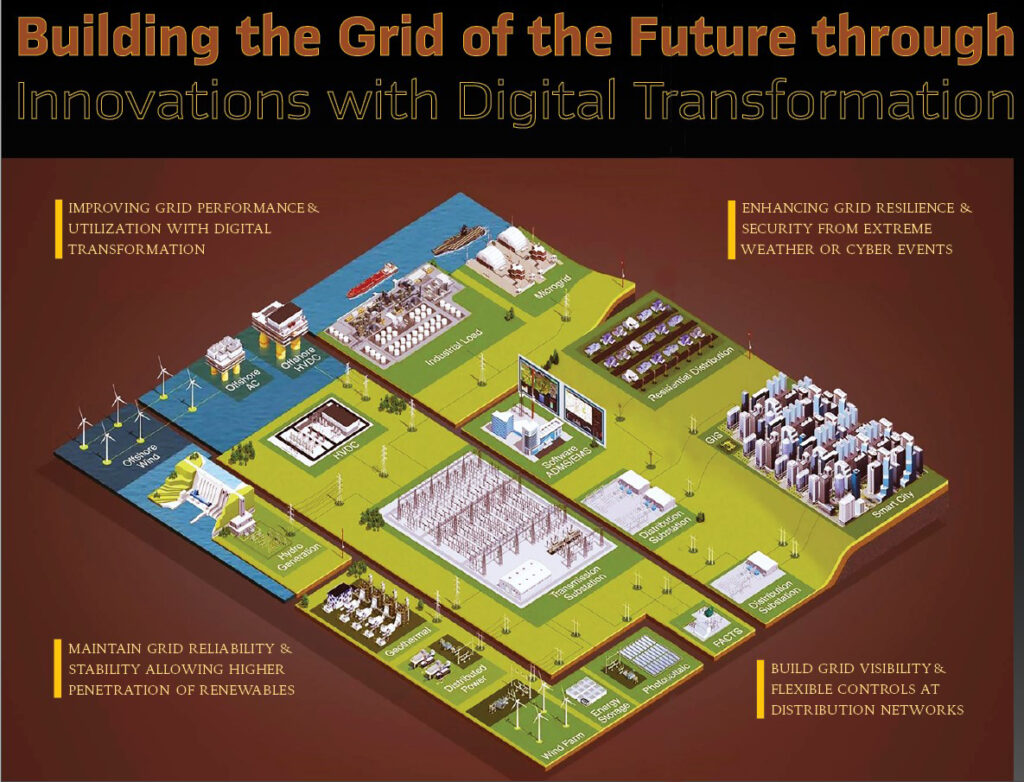
Key drivers of the energy transition that are surveyed across the globe are listed in Figure 2. A higher penetration of renewables at the transmission level with inverter-based resources (IBRs) and distributed energy resources (DERs) at the distribution level have started influencing not only T&D infrastructure planning, but also operational controls and maintenance decisions. There is also increasing demand due to electrification of other industries. The transportation industry for instance, is expected to integrate charging infrastructure to the grid for electric vehicles.
Electric Power Grid – Major Challenges and Opportunities
The electrical grid has been rapidly evolving for more than a century. So, why now? In speaking with many global utilities and heavy power users, three clear factors have emerged as being driving forces: the need and push for decarbonization, the decentralization caused by the growth of renewables and DERs, and digitization–our ability to connect more assets and visualize more data than ever before. Digital transformation enabled by grid modernization technology in the transmission and distribution system will play a central role in the energy transition. Power grid infrastructure has been built and maintained over the past century to deliver reliable, safer, and affordable electricity across communities and industries. With the integration of large renewable energy farms on the transmission grid, and DERs on distribution networks, several challenges and opportunities have presented themselves during the modernizing of decades-old grid infrastructures.
The electric power grid plays a critical role either as an enabler or bottleneck of the energy transition. Address ing the speed and magnitude of the change driven by the energy transition requires the electric power grid to prioritize solutions for: 1) Improving grid performance and utilization with digital transformational solutions, incl. standardized digital substations across T&D; 2) Enhancing grid resilience and security during extreme weather or cyber events; 3) Maintaining grid reliability and stability while allowing a higher penetration of variable renewable energy resources, especially at the transmission network; 4) Building grid visibility and flexibility through distributed controls mainly at the distribution level.
Distributed Intelligence and Control
This section presents a paradigm shift of moving centralized controls to more decentralized distributed intelligence controls.
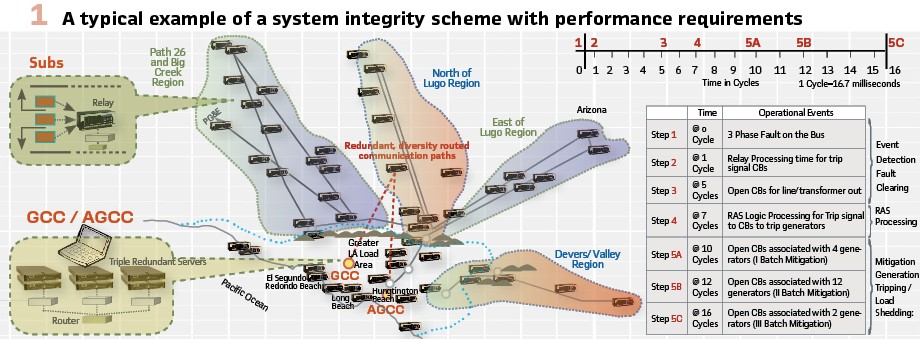
System integrity controls for transmission: System integrity (e.g., stability, and resilience) of the power grid provides fast controls across the transmission and distribution grid, especially with the large integration of low-inertia renewable resources such as those shown in Figure 1. Such system is also known as system integrity protection scheme, special protection schemes, or remedial action schemes. Of the entire system performance budget, three cycles (approximately 50 ms) are allocated for communication and controller/logic latency. Of the 50 ms, 38 ms is designated for communication latency and 4 ms for controller reaction time.
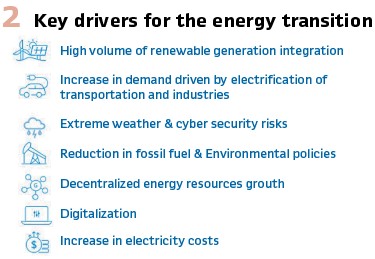
A generic object-oriented system event (GOOSE) mechanism is used for protection and control applications over local area networks. To use GOOSE over WAN for applications, the implementation of routable-GOOSE (R-GOOSE) protocol is used. High-speed (e.g., priority tagging), multicasting, and security mechanisms supported by R-GOOSE use IEC 61850 and IEC 62351 standards. Alternatively, the phasor measurement units and phasor data concentrator-enabled data-driven synchrophasor can be used based on applications, network bandwidth, and performance requirements. For example, the Spanish system includes a submarine high voltage direct current (HVDC) link laid in the Mediterranean Sea that connects the Mallorca Island with mainland Spain. A 244-kilometer-long mass impregnated submarine DC cable connects both converters, one in the Santa Ponsa 220 kV substation, near the island’s capital city Palma de Mallorca, and the other located in the Morvedre 400 kV substation, near Valencia. (Figure 3).
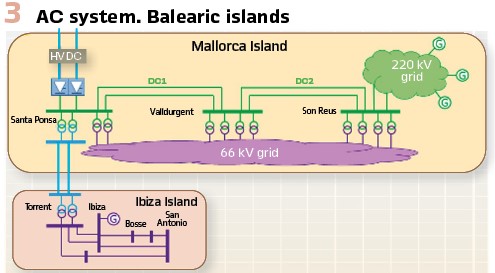
Because of the Line Commutated Converter (LCC) HVDC technology used, the operation of the link requires a minimum short circuit power of 500 MVA in the connection points with the AC network. The amount of power that manages the HVDC system in relation with the total demand in the Balearic system, (approximately 1000 MW,) requires an ultrafast switching of the operation mode in the HVDC link when operating near to its short circuit limits. WAPAC with an Automated Stability Phasor-based Algorithm System (ASPAS) allows for operation optimization with consequent economic benefits. To accomplish the goals, it is critical to have an accurate on-line estimation of the short circuit power at the Santa Ponsa substation. After analyzing different alternatives to estimate the short circuit power, a novel process was decided based on the topology of a nearby network, the measurements of electrical magnitudes in some selected substations, and an algorithm running calculations based on a previous “off-line” big data and statistics methodology analysis. The short circuit power estimation result from the process determined if a change in the operation mode of the HVDC is needed. It is important to note that for this process to be effective it must be done very fast (in less than 70 ms.)
Overall project benefits are as follows:
- Economic: it is not needed to increase the generation in the Balearic system (greater cost than the peninsular one) due to the limitation of the HVDC link.
- Environmental: prevents conventional generation in the Balearic system to cover the limitations imposed by the HVDC, which contributes to a more sustainable Balearic energy mix.
- Technical: after contingencies, prevents the HVDC link can be kept operating with low short-circuit power, which can cause damage to the converter station and the transmission network close to it.
DERs/Microgrid with distributed controls for Distribution: The U.S. Department of Energy Microgrid Exchange Group defines a microgrid as “a group of interconnected loads and distributed energy resources (DERs) with clearly defined electrical boundaries that acts as a single, controllable entity with respect to the grid. A microgrid can connect and disconnect from the grid to enable its operation in both grid-connected or islanded mode.” Or, as I like to say, a self-sufficient island within the larger grid ocean. While the concept and implementation of microgrids are not new, the opportunity now is enabling the energy transition with innovative solutions by utilizing renewable energy. (Figure 4).
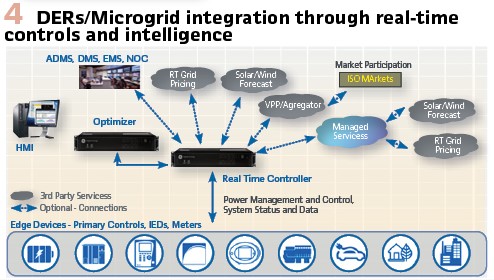
To meet carbon neutrality goals, corporations have started to implement sustainability programs at their facilities. When implemented effectively, these programs involve the integration of onsite renewable energy. Onsite renewable integration varies across a broad spectrum, but when leveraged as part of a microgrid can provide additional benefits beyond sustainability including energy savings, reliability, resiliency, and revenue generation. These benefits are obtained through the integration of advanced control and optimization platforms that are tailored and configured to meet a facility’s objectives. (Figure 7).
- Increased Reliability enhancements through real-time detection of unstable system behaviour and isolation of the affected system ensuring your most critical infrastructure stays online until normal system operation is restored
- Improved Resiliency by providing a system that can self-isolate from the affected grid and continue to support its loads independently for desired periods of time
- Energy Cost Reduction through a solution that can efficiently manage and optimize your energy resources based on real-time energy market prices, operational costs and energy resource mix
- Increased Revenue by enabling your system to provide ancillary services to the grid such as frequency control, reserve capacity, and demand response
- Energy Security by effectively controlling, monitoring and optimizing your local energy assets reducing your need to rely on a centralized supply
- Increased percentage of Renewables Integration through an energy management solution that supports the transition to new renewable energy targets and policies
- Reduced Emissions from on-site generation sources through optimal dispatch and management of your energy resource mix
A distributed control solution is comprised of two parts, the real-time secondary controller and the tertiary level optimizer. The real-time secondary controller is responsible for the reliability of the system through high-speed communication, control, and automation. The tertiary level optimizer is responsible for the customer desired outcome by producing a day-ahead operating or optimal dispatch plan. This plan is tailored based on the facility’s desired outcome or mode of operation such as economics, reducing emissions, maximizing time of life, maximizing renewables, or peak shaving. These two systems work together to provide the facility with an optimal solution to manage the system in either a grid connected or islanded mode, maintaining reliability, resiliency, and customer outcome. (Figure 5).
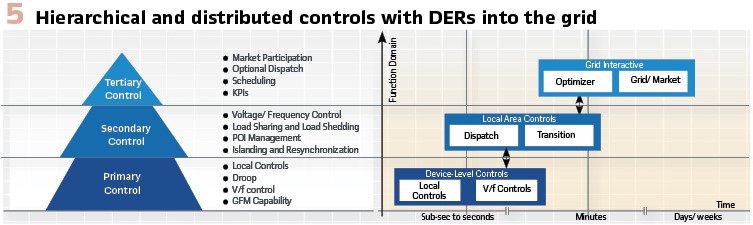
The microgrid real-time controller is responsible for maintaining the reliability of the system, while following the optimal dispatch plan generated by the optimizer. This is achieved through communication with various intelligent electrical devices (IEDs) and the onsite DERs.
Evolving Digital Transformation Across Grid Infrastructures
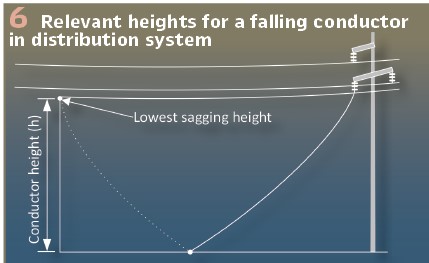
This section presents the digital transformation applied within the substation as well as outside the fence of transmission and distribution networks.
Digital substations – standardization and optimize O&M costs: More and more utilities are investing in new protection, automation, control (PAC) systems in substations. They are doing this for several reasons. First, it allows for a smaller control house footprint which saves money in construction. It also allows for significantly less wiring between devices which lowers cost and reduces the opportunity for miswiring. It also allows for significant data to be available for utilities to use to monitor systems and leverage their asset management programs. The SF6-free technology innovations such as green gas (g3) enabled switchgear already provides clean primary equipment for the grid.
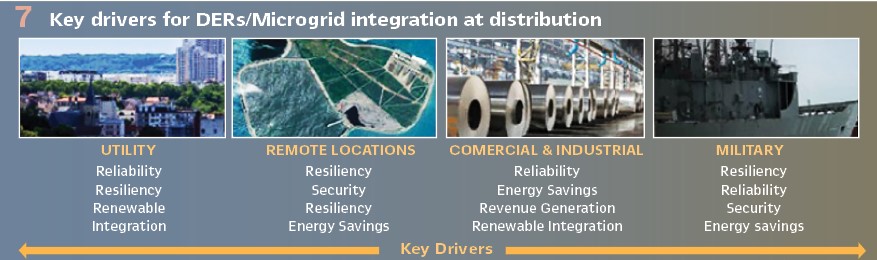
Figure 8 shows the hierarchical architecture of a digital substation PAC system divided into three existing levels: process, bay, and station. Considering the technology cycles discussed earlier, the process level needs to “simplify” through digitization using open standard data sharing communication interfaces (i.e., IEC 61850-9-2LE and/or IEC61869-9/13). The bay level should be able to “converge” PAC applications with centralized substation protection systems. The modularized hardware of PAC IEDs can provide completely independent communication ports to facilitate cyber security perimeter segregation for process bus and station bus. The station bus can “future-proofed” with software-defined applications for the grid of the future. As the grid/substation evolves, the scalable substation control/automation application platform can allow for future applications and solutions without replacing the hardware or platform software.
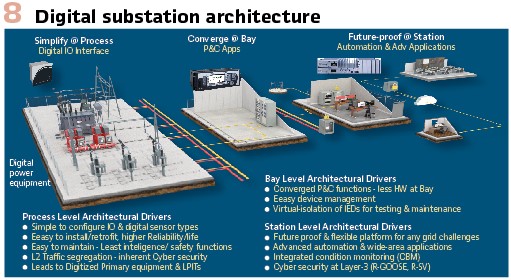
Process interface units installed close to or as part of, primary equipment, are used to digitize the signal interface and communicate to process bus over interoperable IEC 61850-9-2 or IEC 61869-9/13 standards. The software and settings at the process level need to be minimal or, if possible, with minimum settings and segregated layer-2 (ethernet) level traffic. Easy-to-install hardware delivers higher reliability, and, with interoperable protocols, this data would be available to any application at the bay or station level.
Multi-speed of Technologies for a Substation: The historical evolution of protective relaying technology has been significantly influenced by electronics and communication developments. The next generation of digital protective relaying is also receiving direction from evolving technology trends of the industrial internet of things (IIoT), virtualization, digital interfaces of combined optical CT/VT and circuit breakers, as well as digitized primary equipment. Figure 9 illustrates the differences in various technological evolution cycles related to the substation PAC system.
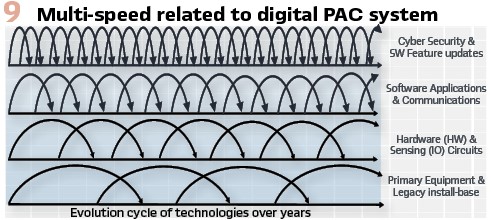
The technologies of primary equipment and legacy system install base cycles around several decades before they are migrated to next generation technology. The lifespan of ruggedized and digitized remote input/output hardware electronics including sensor circuits, can be simplified and stretched to one to two decades. On the other hand, the cycles of software applications and communications technologies are typically evolving at a higher rate (several years); whereas cyber security applications and specific software/firmware may need updates annually or even on demand based on specific requests.
Software-Defined System with Flexibility and Fast Innovation integration: Figure 10 presents innovation driving software-defined grid automation and control platforms. The virtualized architecture may have the potential to migrate non-mission-critical substation automation from native specific hardware platforms to virtual machines or even cloud-native containerized applications.
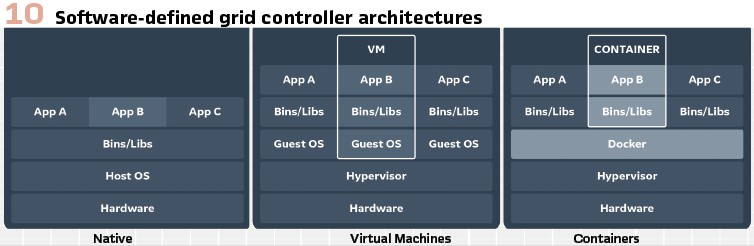
Figure 11 showcases the architecture of an advanced automation application system that allows a real-time operating system with a non-real-time IOT operating system using virtual machine architecture. The hypervisor allows for scalable multi-core CPU cores and the execution of real-time grid logic along with a non-time-critical human-machine interface type of application. The advanced software-defined substation automation system will be using container-based architecture and a secure edge operating system that is lighter than typical virtual machines in terms of footprint and memory usage. Additionally, they boot faster and use fewer resources. They can be deployed seamlessly across development, validation, and production and are easily portable across different computing environments. This system enhances grid reliability and flexibility to adapt to the changes that come with the integration of more renewable energy resources.
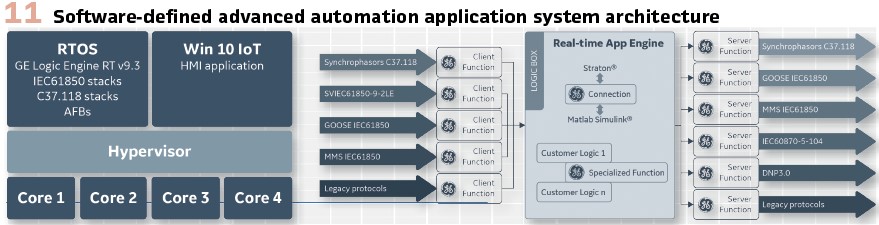
Evolving Grid Analytics from Device & Data Management
Power grid networks and equipment infrastructure are monitored and controlled by edge devices with sensors and actuators with some level of intelligent analytics. As illustrated in Figure 12, the evolution of grid edge analytics moves from a reactive management strategy that responds to failures after they occur to a more autonomous future state with self-healing.
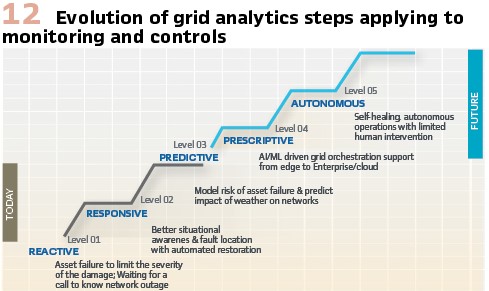
The majority of existing grid edge analytics are reactive to failures to limit damage, with limited responsiveness to restore automatically. The predictive stage may utilize system or asset models with a combination of physics and machine learning methods, including anomaly detection, classifications, and/or learned patterns. Further grid innovations can be applied to suggest potential implications and recommended actions. Finally, an autonomous grid with self-healing would use edge-to-edge communications with very limited or no human interventions.
Grid Safety – Falling Conductor Detection for distribution: An energized overhead power line may break and fall to the ground or on other surrounding objects due to reasons such as severe weather conditions, conductor aging, natural disasters, hardware failures, and/or pole knock-over. When the falling conductor touches the earth or other grounded objects, it may cause a high-impedance (Hi-Z) fault which cannot be detected reliably by conventional overcurrent protection schemes. While current-based algorithms using negative-sequence components can detect most broken conductor faults in transmission systems, their efficiency is compromised in distribution systems. The performance of falling conductor protection schemes in distribution systems depends on several factors such as feeder topology, penetration level of DERs, broken phase location, single-phase switching, and/or protection philosophy (e.g., type of protective devices). The proposed High-Speed Falling Conductor Protection (HFCP) scheme will be able to de-energize the affected circuit prior to the conductor hitting the ground, eliminating the risk of an arcing ground fault or energized circuits on the ground. For this specific system, falling conductor time was calculated around 1.06 seconds, and the system can detect within 200ms, which provides extra time to take other actions, such as blocking auto-reclosing and enhancing the safety of the overall distribution system.
Figure 6 shows the communication architecture within an HFCP solution, the PMU devices located downstream of the substation send associated measurements to the radio master station using a wireless channel. This data is then sent to the RTC to be used by the HFCP algorithm. The internal variables of the HFCP algorithm, including the impedances measured at the location of each PMU device, are also sent to the workstation for monitoring and archiving. The solution can be accompanied by an HMI, which allows the user to interact with the HFCP system and monitor the status of the system.
Grid Edge Remote Device Management: A large amount of grid edge devices are integrated at T&D substations, over the distribution feeder as well as DER/REN sites to protect, control, automate, monitor and diagnose the grid assets and networks. These devices, including IEDs and distribution controllers have hardware and software components that requires life cycle management. Traditionally, the data from these devices is collected locally and manually with individual device vendors’ tools.
New digital tools are required to connect to these devices remotely with multi-vendor interfacing drivers which allow automatic data collection at the substation level, and centralized repository at the enterprise level as shown in Figure 13 This system can potentially reduce the number of field crew visits to local site saving significant OPEX as well as time to manage the assets across geographically dispersed locations.
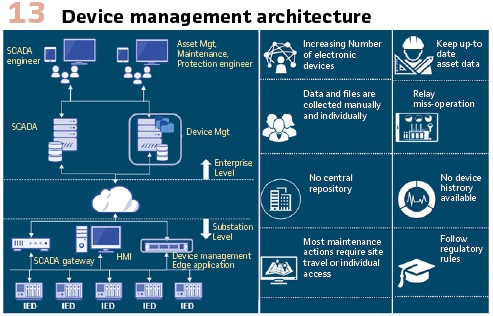
Conclusion: There is no question that grid innovations and digitalization are helping to power the energy transition forward. Globally, the power industry needs more investment in grid modernization and the energy transition to improve grid reliability, safety, sustainability, and affordability. The many parts and pieces of today’s grid infrastructures are insufficient to integrate more renewable resources and maintain supply through extreme external conditions, such as heatwaves or storms.
To address these challenges and unlock sustainable development, utilities are embracing grid innovations and digital transformation across transmission and distribution infrastructures. Enhanced analytics for automatic and online condition monitoring of grid networks and equipment are presented to improve grid reliability/availability and optimize operation and maintenance costs. The digitization of data is integrating previously siloed data streams to provide new visibility into the overall health of critical power system assets. Digital transformation of substation PAC systems with purpose-fit architecture allow convergence and flexibility using international standards for data communication.
Grid resilience and stability are presented with system integrity protection using high-speed secured peer-to-peer communications across the wide-area transmission network. At the distribution level for DER management and microgrid controls can be enabled with recent advancements in industrial-harden communication technology.
The energy transition demands that we continue to modernize the grid to enable the integration of more renewable and distributed energy resources. The developments described in this article are helping while simultaneously entering a new era of clean, reliable, and affordable energy all over the world.
Biographies:

Mital Kanabar is a Senior R&D-Applications Engineering Manager (D&I) at General Electric; and adjunct Professor at the University of Ontario Institute of Technology (UOIT), Canada. He as a Ph.D. from the University of Western Ontario, M.Tech., from the IIT Bombay, India. Mital has 8 years of industrial R&D experience. He is currently vice-chairing the IEEE PES PSRC WG C19 on PDC standard; and WG K15 on centralized substation Protection and Control. He is an active member/contributor of many technical WGs at IEEE PSRC, CIGRE, NASPI, and IEC. He holds 8 international patents and is the author of 40 papers and 3 magazine articles.

Claudia Cosoreanu is Global PM & CTO, Responsible for Grid Automation product strategy and development globally, with the mission to support utility and industrial customers in their transformation journey. Claudia has 20 years’ experience in power and utilities industry with expertise in product management, product development, advanced technologies, strategy, and system thinking.